Nitrous Oxide and California Agriculture
Menu
Management Practices that influence N2O emissions
- Fertilizer Rates
- Fertilizer Placement
- Fertilizer Type
- Fertilizer Timing
- Irrigation Systems
- Nitrification and Urease Inhibitors
- Cover Crops
Frequently Asked Questions
What is nitrous oxide?
Nitrous oxide (N2O) is a powerful greenhouse gas (GHG) produced by microbes that live in the soil. The so-called laughing gas is no laughing matter, as one molecule of N2O released into the atmosphere contributes almost 300 times more to climate change than a single molecule of carbon dioxide (CO2). This means that N2O can have a strong influence on the climate despite being produced in relatively smaller quantities.
Where does nitrous oxide come from?
Nitrification and denitrification are the two main ways that microbes produce N2O. Nitrification and denitrification are both stimulated by nitrogen (N) fertilization.[1]
Nitrification refers to the conversion of ammonia/ammonium (NH3/NH4+) into nitrate (NO3-). This conversion is performed by nitrifying microbes. These microbes consume ammonia/ammonium and “breathe” oxygen. Microbes are not able to convert ammonia/ammonium into nitrate at 100% efficiency, and some N is lost as N2O gas. While overall rates of nitrification decrease as oxygen becomes less available, which can occur following soil wetting or compaction, the amount of N2O produced per molecule of ammonium increases. The nitrification process has two steps: 1) ammonia oxidization, when ammonia is converted into nitrite (NO2-) and 2) nitrite oxidization, when nitrite is converted into nitrate.
Nitrification occurs readily in soils with moderate water contents, as higher levels of soil water limit the amount of oxygen in the soil. However, the relative amount of N2O produced per unit of ammonia processed increases as oxygen levels continue to decrease. This means that significant amounts of N2O can be produced from nitrification even when oxygen levels are low, but above zero. Rising soil temperatures increases the rate of nitrification. Nitrifying microbes prefer a pH between 6.5 and 8.8, so nitrification rates are highest in this pH range.
Denitrification can be thought of as the complementary process to nitrification. Denitrification refers to the conversion of nitrate into nitrogen gas (N2), which is unreactive and harmless in the atmosphere. The term denitrification generally refers to the complete conversion process from NO3- to N2 gas, though individual steps in the process are often referred to as denitrification as well. N2O forms during an intermediate stage of denitrification when, under certain soil conditions, microbes are not able to fully convert nitrate to N2. As N2O is a gas, it can be released from the soil before it has the chance to be converted into N2, especially when it is produced close to the surface. As opposed to nitrifying microbes, denitrifying microbes “eat” carbon containing compounds produced by other microbes and “breathe” nitrate instead of oxygen. In fact, oxygen damages the enzymes used in denitrification, so the process is extremely limited when oxygen is available.
What does nitrous oxide have to do with agriculture?
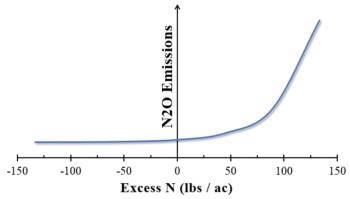
In particular, N fertilization practices contribute significantly to N2O production, as fertilizers increase the availability of ammonia to nitrifying microbes and nitrate to denitrifiers.[3] N2O emissions are highest when fertilizer applications exceed crop needs.
What conditions result in nitrous oxide emissions?
Soil microbes jump into action from the moment that fertilizer is applied to a field, feasting on this new source of nutrients. These microbes will continue to consume the fertilizer nitrogen, and release N2O, as long as it remains available and conditions such as temperature and moisture level allow for continued microbial activity.
The application of ammonia or ammonium based fertilizers results in high rates of nitrification and therefore losses of N in the form of N2O. Application of other N fertilizers, including manure and urea, also associated increase rates of nitrification and N2O production.[4, 5] When availability of ammonia becomes limited, less substrate for nitrification is available and N2O production decreases.
Denitrification occurs when there is available nitrate and water levels from irrigation or precipitation are high enough to expel most oxygen from the soil. For soils that are more compacted, and therefore have less space for air, less water is needed before oxygen levels are low enough for denitrification to occur.[1, 6, 7]
While irrigation and rainfall can result in decreases in soil oxygen, microbes breaking down organic matter also consume oxygen. If enough oxygen is consumed, oxygen levels can be lowered to the point at which denitrification occurs.
When water levels are near saturation, when the soil cannot hold any more water, the main product of denitrification is N2 instead of N2O.[8] Even when this is the case, the denitrification process still represents a loss of N that could otherwise be used by crops.[1]
The rate, timing, source, and placement of fertilizer all impact how microbes process fertilizer N. The relationship between other factors, including irrigation systems and cover crop utilization, also influence N2O emitting microbes.
How can I reduce nitrous oxide emissions without sacrificing returns?
By maintaining maximum fertilizer efficiency, N2O emissions can be limited without reducing yields. Applying fertilizer of the right type, at the right rate, in the right place, and at the right time, also known as the “Four Rs,” ensures that crops receive the nutrients they need while limiting excess nitrogen available to microbes that produce N2O.
N2O emissions can also be reduced by efficient irrigation management, especially the implementation of drip irrigation, which limits the time that conditions ripe for denitrification occur.
A more comprehensive guide on N management can be found on the nitrogen management page. Comprehensive fertilization guidelines for a variety of crops grown in California can be found on this CDFA website.
Continue reading for details about specific management practices that affect N2O emissions.
Fertilizer Rate
Background: Efficient fertilization is the most important way that growers can reduce N2O emissions from their fields. Applying fertilizers beyond what a crop needs increases N that is available to nitrifying and denitrifying microbes, resulting in increased N2O emissions.
Management Considerations: Effective nutrient monitoring is the gold standard of a sustainable growing operation, and limiting N2O emissions is one of many benefits that results from informed fertilization practices.[9] Pre-plant soil nutrient tests help determine appropriate N fertilization rates and are a critical component of developing a nutrient management plan. Pre-sidedress soil nitrate testing “quick tests” can be used to adjust fertilizer application rates mid-season. A detailed nutrient management plan helps to increase fertilizer efficiency and limit N2O production.
Fertilizer Placement
Background: After a concentrated source of N has been added to the soil, microbes are not able to process the added nutrients quickly enough. This can create a backlog of nitrite, which microbes can utilize in place of oxygen, producing N2O in the process. Applying N fertilizer in concentrated bands, as opposed to being broadcast or delivered as part of a drip based fertigation system, therefore stimulates greater N2O losses. With high water levels or low oxygen availability this becomes a significant source of N2O produced from soil.[5]
Management Considerations: Concentrating fertilizer below the soil surface allows for increased plant root uptake.[10] The advantages of increased yields must be balanced with attempts to limit N2O emissions.
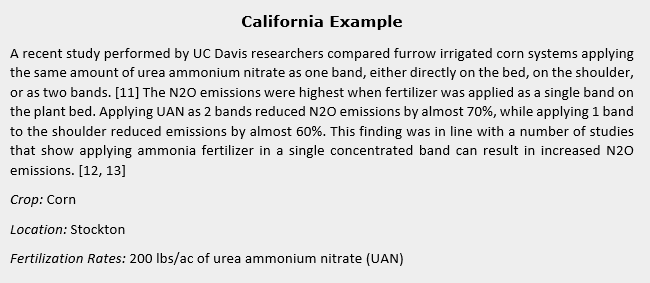
Fertilizer Type
Background: Not all N fertilizers influence N2O emissions in the same way. In particular, ammonia based fertilizers have been shown to stimulate higher N2O emissions than urea based fertilizers in several studies performed outside of California. The application of fertilizers can have a number of effects on soil conditions that influence how N2O producing microbes behave.
Changing the type of fertilizer applied may influence N2O emissions. Anhydrous ammonia, a more concentrated source of N than urea based fertilizer, increases soil pH, which can lead to an increase in soil nitrite and N2O production. Potential differences in fertilizer driven N2O emissions are strongly influenced by soil conditions, such as temperature, moisture, and pH.
Management Consideration: Anhydrous ammonia and aqua ammonia are currently the two least expensive N fertilizer options (as of 2016).
![]() |
![]() |
Other studies performed outside of California also show that ammonia based fertilizers stimulate more N2O emissions than other N fertilizers. [15-18]
Fertilizer Timing
Field studies comparing fertilization timing and N2O emissions have yet to be carried out in California. Research from other locations shows that splitting fertilizer application between two events can reduce N2O emissions by 17-30% without reducing yields.[20, 21]
Management Considerations: For crop specific fertilization guidelines, visit the CDFA fertilization guidelines.
Irrigation Systems
Background: Targeted irrigation strategies, such as subsurface drip and micro-sprinkler systems, can reduce N2O emissions as compared to flood or furrow irrigation.
Two main factors are at play in explaining why targeted irrigation strategies help to reduce N2O emissions. First of all, high levels of soil moisture stimulate N2O production from both nitrification and denitrification. Second, drastic shifts in soil water content cause peak emissions events. In other words, when dry soil gets wet suddenly, either due to heavy irrigation or the first winter rains, a significant amount of N2O can be emitted in a matter of days. Targeted irrigation systems help prevent the conditions ripe for N2O production by keeping the soil consistently moist. Additionally, when N2O is produced deep in the soil near the drip line, there are more opportunities for denitrifying organisms to “catch” N2O and convert it into N2.[22, 23] Furthermore, plant root water uptake can help limit high levels of moisture in shallow soil layers, helping prevent large N2O emissions. [19]
Drip systems are more targeted than furrow irrigation in the delivery of water and nutrients (through fertigation) to crop roots. This can reduce both the time and area over which the conditions that promote N2O production occur. In annual cropping systems, subsurface drip reduces N2O emissions when compared to flood or furrow irrigation. For almond orchards, however, a number of studies suggest that micro sprinkler systems decrease N2O emissions when compared to surface drip.[24] Drip irrigation has also been shown to reduce water use and decrease nitrate leaching. [25]
Management consideration: Implementing drip irrigation has associated costs and specific challenges that must be addressed. For more information about implementing drip irrigation in your cropping system, contact your local UC Cooperative Extension adviser.
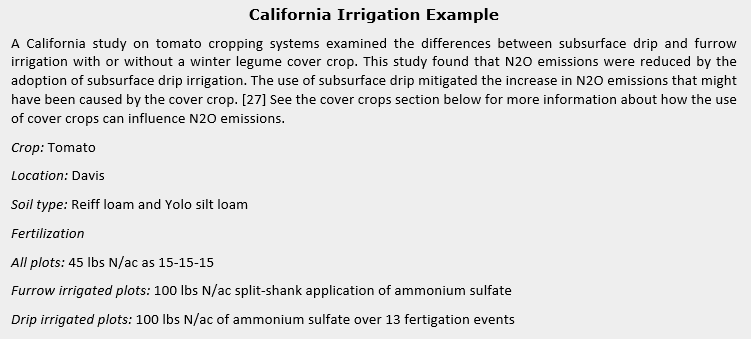
Nitrification and Urease Inhibitors:
Background: Nitrification inhibitors are chemical products that inhibit ammonia oxidation in soil (the first step of nitrification). This limits N2O production from both nitrification, by slowing the rate at which it occurs, and denitrification, by limiting nitrate availability.
Nitrification inhibitors, when applied together with N fertilizer, can reduce N2O emissions by 35%. [35, 36] Several field experiments in California are currently evaluating the efficacy of nitrification inhibitors in reducing N2O emissions.
Urease inhibitors, on the other hand, limit the rate that urea breaks down and becomes available to both plants and microbes. Current research suggests that urease inhibitors are not effective at either reducing N2O production or increasing yields in California. [35, 47]
Management Considerations: Currently dicyandiamide (DCD) and nitripyrin are the only nitrification inhibitors approved in California, but various slow release fertilizers are also being used. To maximize the benefits of nitrification inhibitors, the fertilizer with nitrification inhibitor should be applied as late as possible to maximize crop N uptake as ammonium. For example, a side-dress application should take place when plants are large enough to take up significant amounts of ammonium N. Plant performance may suffer if nitrification inhibitors are applied in excess of their recommended rates.
Cover Crops
The large majority of nitrogen in the atmosphere exists in the form of dinitrogen gas (N2). This gas is unavailable to plants, and must be “fixed” before it can be used. This fixation is performed by a group of microbes known as N-fixers, who convert N2 into ammonia (NH3). Leguminous plants, such as beans or alfalfa, house N-fixing microbes in nodules in their roots. These microbes give some of this fixed nitrogen to plants in exchange for carbon compounds the microbes use as food. This N now stored in plant biomass can become accessible to future crops after the plants are incorporated into the soil, reducing the need for fertilization. However, elevated soil N from legume cover crops can also result in increased N2O emissions.[30] On the other hand, grasses such as ryegrass or millet take up needed N from the soil, potentially limiting losses from nitrate leaching and nitrous oxide production.[31, 32]
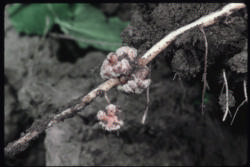
The type of cover crop is a major factor driving differences in N2O production rates. While a recent study summarizing current findings showed that cover crops increased N2O emissions 60% of the time, these observations were split strongly along cover crop species lines. While leguminous cover crops, which introduce additional N to the soil, were frequently found to increase N2O emissions, the use of non-leguminous grasses largely did not cause significant production of N2O. [33] A recent analysis of legume based fertilization systems in Mediterranean climates, like those in California, found that N2O emissions in legume based systems were 60-80% lower when compared to other climate types. [34]
Management considerations: The choice of cover crop and timing of incorporation can be important factors that influence N2O production. Legume cover crops often increase N2O emissions, though the extent of this effect depends on irrigation practices, tillage, and a handful of other factors that are outside the control of growers, including precipitation and soil texture. Incorporating N fertilization credits from leguminous cover crops into nutrient management plans can help reduce N2O emissions stimulated by the use of cover crops. On the other hand, improper management of non-leguminous cover crops can reduce the availability of soil N due to immobilization.
The advantages of cover cropping stand in contrast to concerns about N2O emissions. For more information about the benefits of cover crops, check out the UC SAREP cover crop database and our cover crops page.
Sources
- Kennedy, T., C. Decock, and J. Six, Assessing drivers of N2O production in California tomato cropping systems. Science of the Total Environment, 2013. 465: p. 36-47.
- Snyder, C.S., et al., Review of greenhouse gas emissions from crop production systems and fertilizer management effects. Agriculture, Ecosystems & Environment, 2009. 133(3–4): p. 247-266.
- McSwiney, C.P. and G.P. Robertson, Nonlinear response of N2O flux to incremental fertilizer addition in a continuous maize (Zea mays L.) cropping system. Global Change Biology, 2005. 11(10): p. 1712-1719.
- Davidson, E.A., The contribution of manure and fertilizer nitrogen to atmospheric nitrous oxide since 1860. Nature Geosci, 2009. 2(9): p. 659-662.
- Zhu, X., et al., Ammonia oxidation pathways and nitrifier denitrification are significant sources of N2O and NO under low oxygen availability. Proceedings of the National Academy of Sciences, 2013. 110(16): p. 6328-6333.
- Beare, M.H., E.G. Gregorich, and P. St-Georges, Compaction effects on CO2 and N2O production during drying and rewetting of soil. Soil Biology and Biochemistry, 2009. 41(3): p. 611-621.
- Ruser, R., et al., Emission of N2O, N2 and CO2 from soil fertilized with nitrate: effect of compaction, soil moisture and rewetting. Soil Biology and Biochemistry, 2006. 38(2): p. 263-274.
- Butterbach-Bahl, K., et al., Nitrous oxide emissions from soils: how well do we understand the processes and their controls? Philos Trans R Soc Lond B Biol Sci, 2013. 368(1621): p. 20130122.
- Van Groenigen, J.W., et al., Towards an agronomic assessment of N2O emissions: a case study for arable crops. European Journal of Soil Science, 2010. 61(6): p. 903-913.
- Mengel, D.B., D.W. Nelson, and D.M. Huber, Placement of Nitrogen Fertilizers for No-Till and Conventional Till Corn1. Agronomy Journal, 1982. 74(3): p. 515-518.
- Evaluating Mitigation Options in Nitrous Oxide Emissions in California Cropping Systems. 2016, California Air Resource Board.
- Engel, R., et al., Influence of urea fertilizer placement on nitrous oxide production from a silt loam soil. J Environ Qual, 2010. 39(1): p. 115-25.
- Halvorson, A.D. and S.J. Del Grosso, Nitrogen placement and source effects on nitrous oxide emissions and yields of irrigated corn. J Environ Qual, 2013. 42(2): p. 312-22.
- Zhu-Barker, X., W.R. Horwath, and M. Burger, Knife-injected anhydrous ammonia increases yield-scaled N2O emissions compared to broadcast or band-applied ammonium sulfate in wheat. Agriculture, Ecosystems & Environment, 2015. 212: p. 148-157.
- Fujinuma, R., R.T. Venterea, and C. Rosen, Broadcast urea reduces N2O but increases NO emissions compared with conventional and shallow-applied anhydrous ammonia in a coarse-textured soil. J Environ Qual, 2011. 40(6): p. 1806-15.
- Thornton, F.C., B.R. Bock, and D.D. Tyler, Soil emissions of nitric oxide and nitrous oxide from injected anhydrous ammonium and urea. Journal of Environmental Quality, 1996. 25(6): p. 1378-1384.
- Venterea, R.T., M. Burger, and K.A. Spokas, Nitrogen oxide and methane emissions under varying tillage and fertilizer management. Journal of Environmental Quality, 2005. 34(5): p. 1467-1477.
- Venterea, R.T., M.S. Dolan, and T.E. Ochsner, Urea Decreases Nitrous Oxide Emissions Compared with Anhydrous Ammonia in a Minnesota Corn Cropping System. Soil Science Society of America Journal, 2010. 74(2): p. 407-418.
- Jamali, H., et al., Effect of soil texture and wheat plants on N2O fluxes: A lysimeter study. Agricultural and Forest Meteorology, 2016. 223: p. 17-29.
- Burton, D.L., et al., Effect of split application of fertilizer nitrogen on N2O emissions from potatoes. Canadian Journal of Soil Science, 2008. 88(2): p. 229-239.
- Aita, C., et al., Reducing nitrous oxide emissions from a maize-wheat sequence by decreasing soil nitrate concentration: effects of split application of pig slurry and dicyandiamide. European Journal of Soil Science, 2015. 66(2): p. 359-368.
- Neftel, A., et al., An experimental determination of the scale length of N2O in the soil of a grassland. Journal of Geophysical Research: Atmospheres, 2000. 105(D10): p. 12095-12103.
- Yang, H., et al., Responses of nitrifying and denitrifying bacteria to flooding-drying cycles in flooded rice soil. Applied Soil Ecology, 2016. 103: p. 101-109.
- Schellenberg, D.L., et al., Yield-scaled global warming potential from N2O emissions and CH4 oxidation for almond (Prunus dulcis) irrigated with nitrogen fertilizers on arid land. Agriculture, Ecosystems & Environment, 2012. 155: p. 7-15.
- Sharma, P., et al., Nitrate-Nitrogen Leaching from Onion Bed under Furrow and Drip Irrigation Systems. Applied and Environmental Soil Science, 2012. 2012: p. 1-17.
- Kennedy, T.L., E.C. Suddick, and J. Six, Reduced nitrous oxide emissions and increased yields in California tomato cropping systems under drip irrigation and fertigation. Agriculture, Ecosystems & Environment, 2013. 170: p. 16-27.
- Kallenbach, C.M., D.E. Rolston, and W.R. Horwath, Cover cropping affects soil N2O and CO2 emissions differently depending on type of irrigation. Agriculture, Ecosystems & Environment, 2010. 137(3-4): p. 251-260.
- Tindula, G.N., M. Orang, N., and R.L. Snyder, Survey of Irrigation Methods in California in 2010. Journal of Irrigation and Drainage Engineering, 2011. 139: p. 233-238.
- Aguilera, E., et al., The potential of organic fertilizers and water management to reduce N2O emissions in Mediterranean climate cropping systems. A review. Agriculture, Ecosystems & Environment, 2013. 164: p. 32-52.
- Garland, G.M., et al., Direct N2O emissions following transition from conventional till to no-till in a cover cropped Mediterranean vineyard (Vitis vinifera). Agriculture, ecosystems & environment, 2011. 144(1): p. 423-428.
- Feyereisen, G.W., et al., Potential for a Rye Cover Crop to Reduce Nitrate Loss in Southwestern Minnesota. Agronomy Journal, 2006. 98(6): p. 1416-1426.
- Shipley, P.R., J.J. Meisiner, and A.M. Decker, Concerving Residual Corn Fertilizer Nitrogen with Winter Cover Crops. Agronomy Journal, 1992. 84: p. 869-876.
- Basche, A.D., et al., Do cover crops increase or decrease nitrous oxide emissions? A meta-analysis. Journal of Soil and Water Conservation, 2014. 69(6): p. 471-482.
- Aguilera, E., G. Guzman, and A. Alonso, Greenhouse gas emissions from conventional and organic cropping systems in Spain. I. Herbaceous crops. Agronomy for Sustainable Development, 2015. 35(2): p. 713-724.
- Akiyama, H., X. Yan, and K. Yagi, Evaluation of effectiveness of enhanced-efficiency fertilizers as mitigation options for N2O and NO emissions from agricultural soils: meta-analysis. Global Change Biology, 2010. 16(6): p. 1837-1846.
- Ruser, R. and R. Schulz, The effect of nitrification inhibitors on the nitrous oxide (N2O) release from agricultural soils—a review. Journal of Plant Nutrition and Soil Science, 2015. 178(2): p. 171-188.
- Suddick, E.C. and J. Six, An estimation of annual nitrous oxide emissions and soil quality following the amendment of high temperature walnut shell biochar and compost to a small scale vegetable crop rotation. Science of The Total Environment, 2013. 465: p. 298-307.
- Harter, J., et al., Soil biochar amendment shapes the composition of N2O-reducing microbial communities. Science of The Total Environment, 2016. 562: p. 379-390.
- Angst, T., et al., Impact of pine chip biochar on trace greenhouse gas emissions and soil nutrient dynamics in an annual ryegrass system in California. Agriculture, Ecosystems & Environment, 2014.
- Verhoeven, E. and J. Six, Biochar does not mitigate field-scale N2O emissions in a Northern California vineyard: An assessment across two years. Agriculture, Ecosystems & Environment, 2014. 191: p. 27-38.
- Jackson, L.E., et al., Responses of soil microbial processes and community structure to tillage events and implications for soil quality. Geoderma, 2003. 114(3–4): p. 305-317.
- Jarecki, M.K. and R. Lal, Crop Management for Soil Carbon Sequestration. Critical Reviews in Plant Sciences, 2003. 22(6): p. 471-502.
- Six, J., et al., The potential to mitigate global warming with no-tillage management is only realized when practised in the long term. Global Change Biology, 2004. 10(2): p. 155-160.
- Suddick, E.C., et al., The Potential for California Agricultural Crop Soils to Reduce Greenhouse Gas Emissions: A Holistic Evaluation, in Advances in Agronomy, Vol 107, D.L. Sparks, Editor. 2010. p. 123-162.
- Lee, J., et al., Tillage and seasonal emissions of CO2, N2O and NO across a seed bed and at the field scale in a Mediterranean climate. Agriculture, Ecosystems & Environment, 2009. 129(4): p. 378-390.
- Maltais-Landry, G., et al., Higher flexibility in input N:P ratios results in more balanced phosphorus budgets in two long-term experimental agroecosystems. Agriculture, Ecosystems & Environment, 2016. 223: p. 197-210.
- Hartz, T. K., & Smith, R. F. (2009). Controlled-release fertilizer for vegetable production: the California experience. HortTechnology, 19(1), 20–22.