Soil Health
Introduction
Soil health is the continued capacity of soil to function as a vital living ecosystem that sustains plants, animals, and humans. Oftentimes, the features of a healthy soil are related to the organic matter content.
The soil carbon present in this organic matter is integral to providing the fuel and structure needed to keep a soil healthy. Similar to human health, soil health will vary widely, with different types of challenges arising in different soil types. These challenges are going to differ largely due to the specific mineral composition of the soil,[1], [2], [3], [4], [5] which functions as the “genetics” of a given soil.
What Does a Healthy Soil Do? [6]
The definition of a healthy soil will vary according to the soil’s desired use, but they often incorporate facets of each of the following functions.
- Productivity/yield: healthy soils should be able to maintain yields or, in extreme weather years, minimize yield losses.
- Nutrient cycling: a tight cycling of nutrients, especially fertilizer-derived nitrogen and phosphorus, entails making nutrients available to plants and other organisms as needed, while reducing excess mineralization and nitrification, which can lead to loss of nutrients and adverse environmental effects.
- Holding water for plant uptake: healthy soils have strong soil structure and aggregation, which results in an increased ability of soils to hold plant-available water.
- Filtering contaminants: healthy soils can reduce the amount of contaminants, (e.g. antibiotics or heavy metals) that enter the freshwater supply.[7]
- Withstanding erosion: the increased aggregate stability of healthy soils can help soils to resist erosion and compaction.
- Mitigate crop diseases: healthy soils can lessen the incidence of soil pathogens that might affect crop performance.
Soil Aggregation: the Basis of Soil Health
Many of these methods of improving soil health pertain to the formation and retention of soil aggregates, or clumps of soil particles. As soils receive carbon input, which can be from crop residues, root exudates from live roots, dead roots, or other organic sources (e.g. compost or manure), soil microorganisms work to decompose these materials. In the process, they produce compounds that begin to bind soil mineral particles together into microaggregates. With increasing organic matter inputs these microaggregates begin to bind to one another, forming larger and larger aggregates[8]. Soil carbon within these aggregates is considered “protected” from decomposition from biological activity[9]. Due to changes in the chemical structure of soil organic matter that occurs in microaggregates (aggregates smaller than 250 microns in diameter), the carbon in these microaggregates is generally more protected from decomposition than the carbon in macroaggregates (those larger than 250 microns)[10].
|
As roots decompose, soil microorganisms bind soil mineral particles together. |
Increased soil aggregation from soil organic matter inputs can result in improved soil structure. This improved soil structure results in greater resistance to compaction[11] and increased water retention (especially in sandy and silty soils).[12]
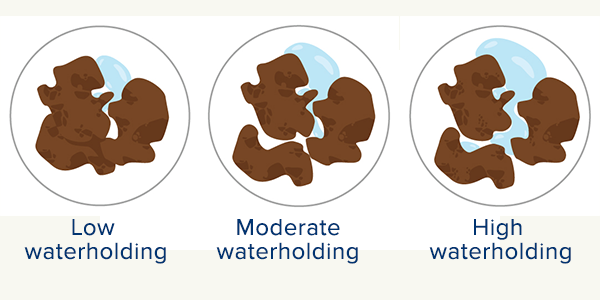
In addition to improving structure, organic matter can increase the stability of soil aggregates when exposed to water[13],[14]. This stability can result in increased water infiltration[15], which means less runoff and erosion[16]. See image below.
![]()
|
Improved soil structure (top) increases infiltration and water holding capacity while decreasing runoff. |
Enhancing Soil Health
1) Use plant diversity to increase soil diversity
As plant roots grow, they exude a variety of carbon-rich compounds that will alter the microbial community composition[17],[18]. Some research suggests that increasing the number of plant species may increase the diversity of the microbial community, which may enhance the soil’s ability to cycle nutrients and supply plant-available N [19],[20].
Additionally, the use of crop rotations has been shown to decrease soilborne pathogens and root diseases. A decrease in pathogens has been linked to increases in soil microbial diversity [21],[22], which can lead to concurrent increases in yield [23]. Currently, it is unclear if increased diversity, absent any pathogen suppression, will increase yields. One recent study showed increased diversity to be associated with higher yields in unfertilized rice fields [24], but it is unclear if this diversity and yield increase persists with fertilization and under other crops.
2) Keep plants growing throughout the year
Plant roots are thought to contribute more carbon to soil than the above-ground biomass [25], although the amount will vary depending on crop and tillage practices [26]. In no-till systems, root-derived carbon is more important than residue-derived carbon [27]. Cover crops are a vital tool to increase both root-derived carbon and total carbon input into a soil. For more information on cover crops, visit our focus topic page or the cover crops database.
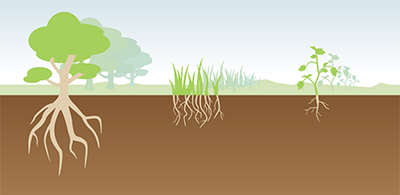
Continuous plant growth throughout the year can help reduce water contamination issues, a vital function for healthy soils to perform. The use of a winter cover crop allows for increased nutrient retention during the rainy months when leaching risk is greatest in California [28]. Perennial crops have also been shown to improve water quality by combating nutrient leaching [29].
3) Manage soils by disturbing them less
Decreasing tillage can increase overall organic matter content [30], although it is often constrained to the top several inches[31],[32]. Tillage decreases soil organic matter by exposing the carbon protected within aggregates to decomposition and loss[33],[34]. This loss of soil carbon can result in increased erosion from water [35] and from wind [36], which is especially important in maintaining air quality in the southern San Joaquin Valley of California.
While no-till practices can result in initial yield reductions, not all studies show this[37], and in some studies yields have generally recovered after a few years and could be mitigated by increasing carbon inputs (through residues, etc.) [38],[39]. Fields that had been cover cropped for 15+ years in California have been shown to have stable or even increased yields from reducing tillage operations [32]. It should also be noted that some California studies have shown that cover crops increase organic matter content more than reducing tillage operations [32],[40].
Tillage, or other similarly disruptive field operations, has also been shown to more strongly alter soil microbial community composition than other variables such as organic versus conventional management [41]. However, most research so far has not shown any definitive effect of tillage on other biological indicators typically used to measure soil health (microbial biomass, respiration, potentially mineralizable N, etc.) [41],[42],[43].
4) Keep the soil covered as much as possible. Soil cover can take several forms, including cover crops and crop residues.
Crop residues can help to maintain yields in no-till systems in dry climates by reducing evaporation and increasing water retention [39]. Soil cover can also help combat erosion [44] in a number of ways. Soil cover, whether from crop residue or other sources of mulch, helps to absorb the impact energy of raindrops falling on soil, reducing soil particle detachment, as well as crusting and sealing of the soil surface.
It also slows the speed of water moving across the surface, reducing the amount of soil particle detachment and transport[45]. Soil cover, whether as standing vegetation or residue, also reduces wind speed at the soil surface, preventing wind erosion. Even a soil cover of only 30% of the soil’s surface can reduce soil loss from wind erosion by 70% [46]. Standing vegetation with some height is more effective at reducing wind speeds than residue lying flat on the surface [46].
Mulch has also been shown to improve biological properties of soil, including earthworm populations and fungal biomass, as well as carbon content[47],[48],[49].
References
1. Denef, K. and Six, J., 2005. Clay mineralogy determines the importance of biological versus abiotic processes for macroaggregate formation and stabilization. European Journal of Soil Science, 56(4), pp.469-479.
2. Fernández-Ugalde, O., Barré, P., Hubert, F., Virto, I., Girardin, C., Ferrage, E., Caner, L. and Chenu, C., 2013. Clay mineralogy differs qualitatively in aggregate-size classes: clay-mineral-based evidence for aggregate hierarchy in temperate soils. European Journal of Soil Science, 64(4), pp.410-422.
3. Six, J., Paustian, K., Elliott, E.T. and Combrink, C., 2000a. Soil structure and organic matter I. Distribution of aggregate-size classes and aggregate-associated carbon. Soil Science Society of America Journal, 64(2), pp.681-689.
4. van Diepeningen, A.D., de Vos, O.J., Korthals, G.W. and van Bruggen, A.H., 2006. Effects of organic versus conventional management on chemical and biological parameters in agricultural soils. Applied Soil Ecology, 31(1), pp.120-135.
5. Doetterl, S., Stevens, A., Six, J., Merckx, R., Van Oost, K., Pinto, M.C., Casanova-Katny, A., Muñoz, C., Boudin, M., Venegas, E.Z. and Boeckx, P., 2015. Soil carbon storage controlled by interactions between geochemistry and climate. Nature Geoscience, 8(10), pp.780-783.
6. Ehmke, T., 2013. Soil health: building for the future. Crops & Soils, 46(1), pp.4-9. (https://www.soils.org/files/publications/crops-and-soils/soil-health.pdf)
7. Huang, W., Peng, P.A., Yu, Z. and Fu, J., 2003. Effects of organic matter heterogeneity on sorption and desorption of organic contaminants by soils and sediments. Applied Geochemistry, 18(7), pp.955-972.
8. Six, J., Paustian, K., Elliott, E.T. and Combrink, C., 2000b. Soil structure and organic matter I. Distribution of aggregate-size classes and aggregate-associated carbon. Soil Science Society of America Journal, 64(2), pp.681-689.
9. Baldock, J.A. and Skjemstad, J.O., 2000. Role of the soil matrix and minerals in protecting natural organic materials against biological attack. Organic geochemistry, 31(7), pp.697-710.
10. Six, J., Conant, R.T., Paul, E.A., and Paustian, K. 2002. Stabilization mechanisms of soil organic matter: Implications for C-saturation of soils. Plant and Soil, 241, pp. 155-176.
11. Hamza, M.A. and Anderson, W.K., 2005. Soil compaction in cropping systems: a review of the nature, causes and possible solutions. Soil and tillage research, 82(2), pp.121-145.
12. Rawls, W.J., Pachepsky, Y.A., Ritchie, J.C., Sobecki, T.M. and Bloodworth, H., 2003. Effect of soil organic carbon on soil water retention. Geoderma, 116(1), pp.61-76.
13. Chenu, C., Le Bissonnais, Y. and Arrouays, D., 2000. Organic matter influence on clay wettability and soil aggregate stability. Soil Science Society of America Journal, 64(4), pp.1479-1486.
14. Kong, A.Y., Six, J., Bryant, D.C., Denison, R.F. and Van Kessel, C., 2005. The relationship between carbon input, aggregation, and soil organic carbon stabilization in sustainable cropping systems. Soil science society of America journal, 69(4), pp.1078-1085.
15. Franzluebbers, A.J., 2002. Water infiltration and soil structure related to organic matter and its stratification with depth. Soil and Tillage Research, 66(2), pp.197-205.
16. Barthes, B. and Roose, E., 2002. Aggregate stability as an indicator of soil susceptibility to runoff and erosion; validation at several levels. Catena, 47(2), pp.133-149.
17. Bais, H.P., Weir, T.L., Perry, L.G., Gilroy, S. and Vivanco, J.M., 2006. The role of root exudates in rhizosphere interactions with plants and other organisms. Annu. Rev. Plant Biol., 57, pp.233-266.
18. Chaparro, J.M., Sheflin, A.M., Manter, D.K. and Vivanco, J.M., 2012. Manipulating the soil microbiome to increase soil health and plant fertility. Biology and Fertility of Soils, 48(5), pp.489-499.
19. Zak, D.R., Holmes, W.E., White, D.C., Peacock, A.D. and Tilman, D., 2003. Plant diversity, soil microbial communities, and ecosystem function: are there any links? Ecology, 84(8), pp.2042-2050.
20. Tiemann, L.K., Grandy, A.S., Atkinson, E.E., Marin-Spiotta, E. and McDaniel, M.D., 2015. Crop rotational diversity enhances belowground communities and functions in an agroecosystem. Ecology letters, 18(8), pp.761-771.
21. Abawi, G.S. and Widmer, T.L., 2000. Impact of soil health management practices on soilborne pathogens, nematodes and root diseases of vegetable crops. Applied Soil Ecology, 15(1), pp.37-47.
22. Garbeva, P., Van Veen, J.A. and Van Elsas, J.D., 2004. Microbial diversity in soil: selection of microbial populations by plant and soil type and implications for disease suppressiveness. Annu. Rev. Phytopathol., 42, pp.243-270.
23. Pankhurst, C.E., Magarey, R.C., Stirling, G.R., Blair, B.L., Bell, M.J., Garside, A.L. and Venture, S.Y.D.J., 2003. Management practices to improve soil health and reduce the effects of detrimental
soil biota associated with yield decline of sugarcane in Queensland, Australia. Soil and Tillage Research, 72(2), pp.125-137.
24. Luo, X., Fu, X., Yang, Y., Cai, P., Peng, S., Chen, W. and Huang, Q., 2016. Microbial communities play important roles in modulating paddy soil fertility. Scientific reports, 6.
25. Rasse, D.P., Rumpel, C. and Dignac, M.F., 2005. Is soil carbon mostly root carbon? Mechanisms for a specific stabilisation. Plant and soil, 269(1-2), pp.341-356.
26. John, B., Yamashita, T., Ludwig, B. and Flessa, H., 2005. Storage of organic carbon in aggregate and density fractions of silty soils under different types of land use. Geoderma, 128(1), pp.63-79.
27. Gale, W.J., Cambardella, C.A. and Bailey, T.B., 2000. Surface Residue–and Root-derived Carbon in Stable and Unstable Aggregates. Soil Science Society of America Journal, 64(1), pp.196-201.
28. Dabney, S.M., Delgado, J.A. and Reeves, D.W., 2001. Using winter cover crops to improve soil and water quality. Communications in Soil Science and Plant Analysis, 32(7-8), pp.1221-1250.
29. Culman, S.W., Snapp, S.S., Ollenburger, M., Basso, B. and DeHaan, L.R., 2013. Soil and water quality rapidly responds to the perennial grain kernza wheatgrass. Agronomy Journal, 105(3), pp.735-744.
30. Six, J., Elliott, E.T. and Paustian, K., 1999. Aggregate and soil organic matter dynamics under conventional and no-tillage systems. Soil Science Society of America Journal, 63(5), pp.1350-1358.
31. Álvaro-Fuentes, J., Plaza-Bonilla, D., Arrúe, J.L., Lampurlanés, J. and Cantero-Martínez, C., 2014. Soil organic carbon storage in a no-tillage chronosequence under Mediterranean conditions. Plant and soil, 376(1-2), pp.31-41.
32. Mitchell, J.P., Shrestha, A., Horwath, W.R., Southard, R.J., Madden, N., Veenstra, J. and Munk, D.S., 2015. Tillage and cover cropping affect crop yields and soil carbon in the San Joaquin Valley, California. Agronomy Journal, 107(2), pp.588-596.
33. Rovira, A.D. and Greacen, E.L., 1957. The effect of aggregate disruption on the activity of microorganisms in the soil. Crop and Pasture Science, 8(6), pp.659-673.
34. Reicosky, D.C., Dugas, W.A. and Torbert, H.A., 1997. Tillage-induced soil carbon dioxide loss from different cropping systems. Soil and Tillage Research, 41(1), pp.105-118.
35. Montgomery, D.R., 2007. Soil erosion and agricultural sustainability. Proceedings of the National Academy of Sciences, 104(33), pp.13268-13272.
36. Madden, N.M., Southard, R.J. and Mitchell, J.P., 2008. Conservation tillage reduces PM 10 emissions in dairy forage rotations. Atmospheric Environment, 42(16), pp.3795-3808.
37. Roper, M.M., Gupta, V.V.S.R. and Murphy, D.V., 2010. Tillage practices altered labile soil organic carbon and microbial function without affecting crop yields. Soil Research, 48(3), pp.274-285.
38. Minoshima, H., Jackson, L.E., Cavagnaro, T.R., Sánchez-Moreno, S., Ferris, H., Temple, S.R., Goyal, S. and Mitchell, J.P., 2007. Soil food webs and carbon dynamics in response to conservation tillage in California. Soil Science Society of America Journal, 71(3), pp.952-963.
39. Pittelkow, C.M., Liang, X., Linquist, B.A., Van Groenigen, K.J., Lee, J., Lundy, M.E., van Gestel, N., Six, J., Venterea, R.T. and van Kessel, C., 2015. Productivity limits and potentials of the principles of conservation agriculture. Nature, 517(7534), pp.365-368.
40. Veenstra, J.J., Horwath, W.R. and Mitchell, J.P., 2007. Tillage and cover cropping effects on aggregate-protected carbon in cotton and tomato. Soil Science Society of America Journal, 71(2), pp.362-371.
41. Bossio, D.A., Scow, K.M., Gunapala, N. and Graham, K.J., 1998. Determinants of soil microbial communities: effects of agricultural management, season, and soil type on phospholipid fatty acid profiles. Microbial ecology, 36(1), pp.1-12.
42. Jackson, L.E., Calderon, F.J., Steenwerth, K.L., Scow, K.M. and Rolston, D.E., 2003. Responses of soil microbial processes and community structure to tillage events and implications for soil quality. Geoderma, 114(3), pp.305-317.
43. Shestak, C.J. and Busse, M.D., 2005. Compaction alters physical but not biological indices of soil health. Soil Science Society of America Journal, 69(1), pp.236-246.
44. Zuazo, V.H.D. and Pleguezuelo, C.R.R., 2008. Soil-erosion and runoff prevention by plant covers. A review. Agronomy for sustainable development, 28(1), pp.65-86.
45. Shelton, D., Jasa, P., Brown, L., Hirschi, M. 2000. Water erosion. Ch. 4 in Reeder (ed.) Conservation Tillage Systems and Management. MWPS-45. Midwest Plan Service, Iowa State University, Ames, Iowa. Pp 17-21.
46. Lyon, D., Smith, J., Fryrear, D. 2000. Wind erosion. Ch. 3 in Reeder (ed.) Conservation Tillage Systems and Management. MWPS-45. Midwest Plan Service, Iowa State University, Ames, Iowa. Pp 11-15.
47. Blanco-Canqui, H., and R. Lal. 2007. Impacts of long-term wheat straw management on soil hydraulic properties under no-tillage. Soil Science Society of America Journal 71:1166-1173.
48. Kahlon, M.S, R. Lal, and M.A. Varughese. 2013. Twenty-two years of tillage and mulch impact on physical characteristics and carbon sequestration. Soil and Tillage Research 125:151-158.
49. National Soil Survey Center (NSSC). 2015. Soil Health Literature Summary – Effects of Conservation Practices on Soil Properties in Areas of Cropland. USDA: Natural Resources Conservation Service, Washington, DC.
50. West, T.O. and Post, W.M., 2002. Soil organic carbon sequestration rates by tillage and crop rotation. Soil Science Society of America Journal, 66(6), pp.1930-1946.