Contact Us
University of California Cooperative Extension Ventura County
669 County Square Drive, Suite 100
Ventura, CA 93003
Phone: 805.645.1451
Fax: 805.645.1474
Office Hours:
Monday - Friday from 9 a.m. to 4 p.m.
The office will be closed for the following holidays:
Soil and Water Management - 2001
From rocks come soils. All soils were once part of rock, but with time and various physical and chemical processes of weathering, rock becomes unconsolidated into loose rock particles. It is from these particles that a soil may develop; it is called parent material.
The transformation of rock into soil is called soil formation and can be shown as follows:
Rock-->weathering-->parent material-->soil formation-->soil
The process of soil formation releases nutrients for plant growth that have been tied up in the minerals of the rocks. The properties of individual soils are closely related to the properties of the parent material from which they have been derived. Knowing the parent material can tell much about the soil, since the parent material will determine the amount and kind of nutrients present, the texture and various other physical and chemical properties.
Parent material has various effects on the rates of soil formation. For example, in a sandy-textured soil, lime (CaCO3) will leach more rapidly than from a fine-textured soil, causing the soil to acidify. Frequently a fine-textured soil has a greater nutrient capacity and more nutrients than a coarse-textured one. Organic matter in the soil is also increased, due to the greater plant growth resulting from more plant nutrients. Fine texture is usually reflected in a greater water holding capacity of the soil, as well.
The chemical composition of the parent material has a substantial effect on the nature of the soil. For example, young soils derived from limestone often have a much higher level of plant nutrients than a young soil from a granitic origin. This higher level of nutrients is going to result in greater plant growth which in turn will affect the rate of soil formation.
The weathering or soil-forming process is a result of climate (rain and temperature), organisms (microbes, plants, animals), relief (topography), parent material, and time. The interaction of these processes has been titled the "CLORPT Equation" by the late Hans Jenny of U.C. Berkeley. In some environments one of the factors may be more important than others. For example, on a steep slope, soil may be thin because it is constantly eroding, but at the base of the slope soil, the soil may be quite deep because rain has had time to act on the material in place.
When speaking of the age of a soil, it is not time necessarily that "ages" a soil.. The age of a soil is relative. Age is how transformed from the original a soil is. As soil becomes older, it develops distinct layers or horizons. The horizons are labeled with letters to distinguish them. The top horizon, commonly called topsoil, is given the letter "A." This letter indicates a layer of mineral soil which has been enriched by the accumulation of organic matter derived from plants growing in this layer. This is the zone of greatest biological activity.
Below the "A" horizon, frequently exists a soil layer where clay has accumulated by migration from the "A" horizon. This horizon of clay accumulation is called the "B" horizon. In young soils where there has been insufficient time for clay to accumulate, there is no "B" horizon present. As soils age, increasing amounts of clay accumulate until a "B' horizon forms. If the soil remains stable for tens of thousands of years, enough clay can accumulate to result in dense, growth-restricting layer called a claypan.
The layer of loose soil beneath the "A" or "B" horizons, which is largely unaffected by organic matter or clay accumulation, is called the "C" horizon. The "C" horizon is often called the parent material of the overlying horizons.
Soils which form in hilly or mountainous terrain usually have a consolidated rock below the soil horizons. The rock layer is termed the "R" horizon, or regolith. If the depth to consolidated rock is deeper than 6 or 7 feet, the soil is usually considered to lack an "R" horizon.
In principle, as soon as a surface is available for plant growth, it becomes a soil, but the soil formation process will continue indefinitely until it is covered by more sediment or is eroded. The soil around houses has often been disturbed by development, and may not show a neat profile of soil formation. What is at the surface may not even be from the site. The best places to see soil horizons are at road cuts or where excavation is being done.
Figure 1. Each horizon has different chemical, physical and biological features which distinguish one from another.
Countering the forces of soil formation are the forces of erosion - wind, water, gravity and in certain areas of the country, snow and ice. As soon as erosive forces abate, soil formation commences.
Soil Physical Properties
The physical properties of soils affect many properties of plant growth and soil management. These properties are texture, structure, density, porosity, consistency, soil organic matter and color. Having an understanding of these properties can help in soil fertility management.
Texture
Soil texture is the relative proportions of sand, silt and clay in a soil. In some cases, larger mineral fractions predominate and a gravely or sandy soil results. In other cases, the finer or colloidal (small enough to stay in suspension) fraction predominates and the soil has clayey characteristics. A sandy soil is often called a "light" soil, while a clayey soil is referred to as a "heavy " soil. These terms are only a reflection of the relative ease of working the two soils. While organic matter may have a tremendous affect on a soil's physical properties, it is not normally considered in a soil's texture because its can vary so greatly.
Soil is a collection of solid material (including organic matter), water and air. The percentages of solids, water and air differ according to the soil's texture. The percentages for a moist, loam soil are:
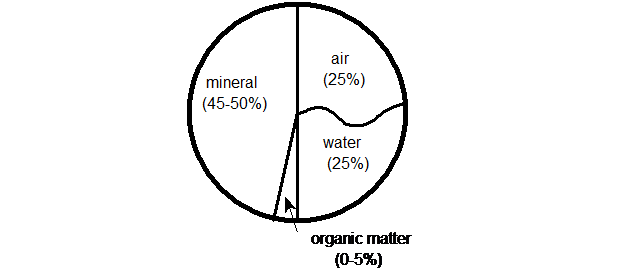
The reason for assessing soil texture is that physical properties will change according to the proportions of each size fraction (or soil separate) present. Properties change largely due to the surface area of the particles. A soil of clay particles has about 10,000 times the surface area of a soil of sand grains of the same weight.
As surface area increases due to more clay particles, the soil becomes more sticky and plastic (able to be molded). Additionally it shrinks and swells, holds more water, has slower gas and water movement and generally holds more nutrients. Sandy soils have a lower water-holding capacity, but, as a result of their larger pores, have better water drainage and air movement.
Soil scientists give names to different textures of soils based on the proportions of sand, silt and clay in the soil. There are 13 textures which can be used to describe soils, and often these are grouped into larger categories (Table 1). By using the "Feel method" (Fig.2), one can easily arrive at a soil's texture.
Table 1. Soil textural classes. | ||
General
|
Terms
|
Texture
|
sandy (light)
|
coarse
|
sand
|
loamy sand
|
||
loamy
|
moderately coarse
|
sandy loam
|
fine sandy loam
|
||
medium
|
loam
|
|
silt loam
|
||
silt
|
||
moderately fine
|
clay loam
|
|
sandy clay loam
|
||
silty clay loam
|
||
clayey (heavy)
|
fine
|
clay
|
sandy clay
|
||
silty clay
|
Bulk Density
What is heavier, a pound of cement or a pound of feathers? They're the same, but what is different? The volume of each material needed to obtain a pound. This is the basis of bulk density. Generally, rocks have a density of 2.65 grams per cubic centimeter (g/cm3 or weight/volume). There is some variation depending on the minerals present, but for most purposes a solid piece of rock has a bulk density 2.65 g for every cubic centimeter of volume. If this solid rock is crushed, some of the volume will now be air along with the rock, and the bulk density decreases.
The more finely the rock is crushed, the lower becomes the bulk density because there is more and more air occupying a given volume. The voids between the particles are called pores, so that, as the material is crushed more and more finely, the total porosity increases. This is analogous to filling a coffee grinder to the brim with beans, grinding the beans and then finding the ground coffee spilling out of the grinder because the coffee's volume has been expanded.
As the porosity increases, the amount of surface area increases. As soil particles become smaller and smaller, the soil has more surfaces. This is an important concept to remember when water and soil fertility are discussed.
If porosity increases with decreasing particle size, why does a sandy soil (higher bulk density, lower porosity) drain better than a clayey soil? The clay soil may have more total pores, but they are much smaller than the large pores of sand and are less able to transmit both water and gasses.
Compaction confounds the native bulk density of a soil. When pressure (foot, truck, fallen tree, etc.) is placed on soil (especially wet soil), the pore space is compressed and the volume reduced, increasing the density. Undisturbed sandy soils generally have a higher bulk density than a clayey soil, e.g.,1.6 vs. 1.2 g/cm3. However, sandy soils are more resistant to compaction than a finer textured soil. A compacted, sandy soil of the same moisture as a clay soil may only increase its bulk density to 1.8, but the clay may increase to 2.0. A surface soil will often have a higher bulk density than the subsurface because it has been compacted. Especially in hilly lands, a compacted surface leads to lower water holding capacity and infiltration rate, which can lead to more rainfall runoff and erosion. One reason for cultivating, is to reduce the bulk density of soil to make it easier for plant roots and water infiltration. After turning the soil, walking on it should be avoided. If necessary put down a board and walk on it.
The presence of organic matter can have a tremendous effect on bulk density. Organic matter has a low bulk density and will decrease the bulk density of a soil, as well as changing other physical and chemical soil properties as it is added.
Soil structure
Knowing the proportions of sand, silt and clay present tells us soil texture. Soil structure tells how these particles are arranged. The grouping of particles in different arrangements plays a very important part in such physical properties as water movement, aeration, bulk density and porosity, just as texture does. A soil of "good" structure will have good drainage, infiltration, aeration and overall good tilth.
Different cementing agents, such as clays, humus, plant and microbial exudates, silica and carbonates bind different soil particles into aggregates or peds. Aggregates are naturally occurring structures that take on characteristic shapes reflective of individual soils. These shapes can be flat (platy), columnar, cubical (blocky), or crumby. The shapes can be large or small, well-defined or poorly-defined. They can be nonexistent (structureless or massive). The degree of development of structure is enhanced with the amount of clay and organic matter present. If a form is artificially created (by tillage or digging) it is called a clod.
The formation of aggregates is due to the various forces in the soil which push particles together: earthworm activity, root growth, shrink/swell on wetting and drying, freezing/thawing. Climate is very important as it affects rainfall, temperature, types of plants growing and, therefore, the amount of organic matter present in the soil. The presence of different salts of calcium in the soil contributes to aggregation, as well. Sodium tends to disperse soils (deflocculate aggregates), destroying structure.
Soil structure takes years to form, and can be instantly lost through mismanagement. Working or walking on soil when it is wet leads to compaction and loss of structure. Soil needs to be somewhat moist to work it, but wetting s soil and immediately working it will ruin it. A soil should be allowed to drain for one to two days after watering it before working it. The length of time depends on the soil texture, the more clay, the longer the time.
A soil profile may have a single type of aggregation, but more commonly there is a characteristic structural pattern with each horizon. This will be determined in large part by the degree of weathering of the soil, which in turn is a function of the soil forming factors: climate, parent material, time, relief and organisms present.
Soil color
Soil color is a helpful guide to the condition of the soil. For example, the darkness or lightness of the soil will influence the temperature of the soil, light soil being cooler, dark, warmer. Color is a function of the amount of organic matter present, the color of the original parent material, the degree and kind of weathering of the soil, the amount and type of surface salts present and the aeration of the soil. Organic matter imparts a darkness to the soil and, in part, indicates the fertility of the soil. The soil's darkness can also be due to the parent material (PM); a light soil may be from a siliceous (silica containing) PM which would have a lower inherent fertility than a darker mafic (high in magnesium and iron) PM. As a soil ages, its color gradually turns red or yellow, which indicates that it is losing nutrients. In arid or poorly drained environments, salts can accumulate at the surface, usually calcium carbonate, calcium sulfate or sodium carbonate. These salts are normally white, but if there is any organic matter in the soil, the sodium salt will cause oxidation (slow combustion) of the material, turning it black, as well as causing aggregates to disperse. Within the soil, if there are periods of poor aeration due to poor drainage, mottles will appear, usually rust colored. If there is prolonged water logging, gleying (blue, green or gray colors) will appear . Just looking at soil color can tell many potential problems with that soil.
Soil organic matter
The organic fraction of soil includes living and dead organic matter. The dead organic matter is in various stages of decomposition, and when the material becomes resistant to further decomposition it is called humus. Soil humus is very important to soil fertility. It has the ability to retain plant nutrients (like clay), and can aid in the formation of aggregate structure. The living portion of organic matter is also important in soil fertility. Earthworms help to maintain good soil physical properties. Bacteria, fungi and other microorganisms are required for humus formation, and they often aid plants in obtaining essential nutrients. Some of the soil microorganisms are very detrimental to plant growth, but careful irrigation management can control many of these pathogens.
Since organic matter is important to soil fertility, it is often added to garden soils in large amounts. An important consideration in the addition of organic matter to soils is the relationship between the amounts of carbon and nitrogen in the organic matter. Some plant material, such as straw or bark has a high carbon to nitrogen ratio. This high C:N ratio will slow the decomposition of the straw and if there is any available nitrogen in the soil, the nitrogen will be absorbed by the microbes decomposing the straw. Since microbes can absorb nitrogen faster than plants, there may be an induced nitrogen deficiency if high C:N materials are incorporated just prior to planting or during plant growth. If high C:N materials are merely applied to the surface as a mulch, nitrogen deficiency is not a problem, and can be a real benefit in reducing weeds and retaining soil moisture.
Composting is an excellent method of obtaining organic matter to add to soil. There are some important aspects of composting that must be considered if the use of compost is contemplated. The most important factor is time. Depending of the level of management, fresh organic matter can become compost in as little as 2 or 3 weeks. With little management, it can take two to four warm months, or five to seven cool months. The length of time will depend on the composition and condition of the materials in the pile, the more ideal the constituents, the faster decomposition will proceed. The general rule of thumb is that the pile needs to be warm, aerated, moist and comprised of about equal proportions of chopped green, fresh material (e.g., grass, kitchen scraps, etc.,) and dry, brown materials (e.g., straw, paper, dry leaves, etc.). And contrary to what you may have heard, eucalyptus and oleander can go in a compost pile. The end-products of decomposition for all plant materials are minerals, CO2 and H2O. Decomposition will handle any potential toxins.
An important factor in the use of composts is the potential spread of weed seeds and plant diseases. Spread of weed seeds is controlled by excluding seed bearing weeds from the compost pile. The disease problem can be controlled by insuring that the organic matter is well decomposed before it is added to the garden. If adequate (>102-140° F) temperatures are sustained in the pile, most weed seeds and diseases are controlled. For further information on composting, see the reference list at the end of this paper.
Management of soil physical properties
Depending on the intensity of management and the resources available, there are many ways of influencing soil physical characteristics. Addition of organic matter to both heavy and light soils can improve aeration and moisture conditions principally by improving aggregation, porosity and bulk density. This may be impractical on large areas or for low profit operations, simply due to the expense, volume and unavailability of material. In this case, conservation of soil organic matter can be practiced by reducing erosion (most organic matter is in the surface horizon of mineral soils) and ensuring that the soil is covered with growing vegetation as much of the year as possible. The detrimental effects of compaction and loss of structure can be averted by reducing traffic, designating traffic areas and by not working the soil when it is wet. The detrimental effects of salts and poor drainage/aeration can be addressed by various reclamation and engineering solutions, expense being the major criterion of which method to use.
Soil Chemistry
It may be hard to imagine, but the soil is one giant, complex biochemical reaction. As organic matter decomposes and soil particles dissolve, materials are released. These can be valuable plant nutrients (Table 2) or, if in high enough concentration, can be toxic materials, such as salts, sodium, chloride and boron. These ions are invariably charged, meaning they have a positive(+) or negative (-) charges on them. These are called ions. Just as clay soils hold more water than sandy ones, a clay will hold more positively charged ions because a clay soil has more surface area to attract ions. Soils in most cases are negatively charged, so that negatively charged ions, such as chloride and sulfate are repelled by soils. This means they are easily leached from soils with adequate irrigation or rain. Soil organic matter is also negatively charged; therefore, it can act to hold nutrients, as well.
Soil pH is another element of soil chemistry that needs to be considered in addition to the presence of nutrients in the soil. The more hydrogen ion (H+) present in the soil system, the more acid it is. The less hydrogen, the more alkaline the soil. The pH range is from 1 to 14, with 14 being the most alkaline. A value of 7 is neutral, neither too acid nor alkaline. The closer to pH 1, the more acid. Most soils in California range from pH 5 to 8.5.
Acidity and alkalinity are important because plants usually thrive in a given pH range. The more acid a soil, the more micronutrients are available in the soil (such as iron, zinc and manganese), and the less calcium and phosphorus are available. Increasing alkalinity much above pH 7 can have the reverse effect of decreasing micronutrients and increasing calcium and phosphorus. A near-neutral or just slightly acid (pH 6.5) soil is considered ideal for most plants, since most nutrients are available around pH 7. There are some plants that prefer a more acid soil, such as azalea or blueberry, but instead of trying to amend the soil to lower the pH, the best solution is to plant something that is adapted to that soil's pH.
Adjusting soil pH can be done with lime (calcium carbonate) or wood ashes when the pH is low, and with sulfur when the pH is high. The materials need to be incorporated in the rooting area of the soil. Soil pH can change soon after watering the soil for lime, but may take several months for sulfur to lower pH. This is because sulfur conversion to acid is mediated by microbes which take their time and is temperature and moisture dependent. Long-term use of ammonium fertilizers also has a tendency to lower pH. And because one of the breakdown products of organic matter is ammonium and carbon dioxide (carbonic acid when it is dissolved in water), additions of various organic materials tend to lower pH's of high pH soils.
A common misconception is that gypsum lowers soil pH. The major effect of gypsum is that of improving water infiltration. If a sodium salt problem is present (which can cause high pH), by increasing infiltration and leaching of the sodium, soil pH will decrease. If a sodium condition is not present, gypsum will not lower soil pH. If the soil is strongly resistant to pH change after additions of soil amendments, specific fertilizers can be applied to plants, such as iron sulfate or chelate to correct plant iron deficiency symptoms.
Table 2. Essential Mineral Elements for Plants. | |||
Element
|
Symbol
|
Form Available
to plant |
Sourse
|
Hydrogen |
H
|
H2O
|
Water
|
Carbon |
C
|
CO2
|
Air
|
Oxygen |
O
|
O2,H2O
|
Air, Water
|
Nitrogen |
N
|
NO3-,NH4+
|
Soil, Air
|
Phosphorous |
P
|
H2PO4-
|
Soil
|
Potassium |
K
|
K+
|
Soil
|
Calcium |
Ca
|
Ca+2
|
Soil
|
Magnesium |
Mg
|
Mg+2
|
Soil
|
Sulfur |
S
|
SO4-2
|
Soil
|
Iron |
Fe
|
Fe+3
|
Soil
|
Boron |
B
|
H3BO3
|
Soil
|
Zinc |
Zn
|
Zn+2
|
Soil
|
Manganese |
Mn
|
Mn+2
|
Soil
|
Chlorine |
Cl
|
Cl-
|
Soil
|
Copper |
Cu
|
Cu+2
|
Soil
|
Molybdenum |
Mo
|
MoO4-2
|
Soil
|
Nickel |
Ni
|
Ni+2
|
Soil
|
Hunger Signs in Plants
Since Greek and Roman times, the appearance of a plant has been used to help identify plant health. The plant speaks through distress signals. The message may be that there is simply too little or too much water. Or the sign may tell us of a disease caused by a microorganism, such as a fungus, virus or bacteria. The plant may show symptoms of attack by nematodes, insects or rodents or from injuries from frost or lightning. According to the plant species, these signals may differ slightly, but frequently they can be generalized.
It is also possible to generalize about the signals linked to the nutritional status of a plant. Learning these symptoms can alert us to appropriate steps to correct the toxicity, deficiency or imbalance of nutrients.
There are 17 elements essential for plant growth (Table 2). Hydrogen, oxygen, and carbon come either from the air or water. The others come from the soil. Depending on the quantity needed by the plant, these are called either primary or trace (micronutrients) nutrients. The micronutrient nickel is required in such small amounts (50-100 parts per billion) by plants that it was identified only in 1990 as being an essential nutrient. Other micronutrients are iron, manganese, boron, chlorine, zinc, copper and molybdenum. Some other nutrients have been identified as being essential for only certain plants, such as silicon for sugar cane.
The primary nutrients are measured on a percent (parts per 100) dry weight tissue basis. These are nitrogen, phosphorus, potassium, calcium, magnesium and sulfur. The trace elements are measured on a part per million dry weight basis. For example, a typical analysis of a dried leaf from a healthy tomato plant might show 3% nitrogen, 1% potassium, 100 ppm (parts per million) iron and 50 ppm boron.
Although plants require more primary than trace nutrients, all the essential elements need to be present for a healthy plant. An excess, deficiency or even an imbalance of these elements will lead to individual symptoms which are characteristic to most plants. The following list is a description of the more common nutritional problems in California:
Excess or toxicity
- Boron - chlorosis (yellowing), leading to tissue death (necrosis) along the margins of older leaves.
- Sodium , Chloride - necrosis of the leaf tips and margins on older leaves.
Deficiency
- Phosphorus - smaller plants; occasionally, older leaves darker than normal or have a reddish or purplish cast, a common symptom in sweet corn.
- Potassium - scorching (firing or necrosis) along leaf margins of older leaves; plant growth slow, with poorly developed root system; stalks weak and fall over.
- Nitrogen - older leaves chlorotic, venation not prominent as in iron deficiency.
- Zinc - depending on the plant species, occasionally interveinal (between the leaf veins) chlorosis on younger leaves, but frequently the leaves small and in a rosette (leaves clustered in a circular pattern near branch tips).
- Iron - younger leaves will show interveinal chlorosis.
These and other problems can be corrected with appropriate fertilizers, amendments and manures and also by soil and water management. Not enough can be said about appropriate water management, especially if it is of a poor quality (high in salts, chloride , sodium of boron). When a plant transpires water through its leaves, any salts that were in the water are left behind. These can gradually accumulate and cause toxicities if there is inadequate rainfall or enough water is not applied to leach these salts below the root zone. In well-managed plants, you may never see the symptoms discussed here, but learning the signals can help direct your activities if you do.
There are some excellent publications with photographs that can be obtained at nurseries and libraries that will aid in identifying the hunger signs of plants. If it is vital that a nutrient problem be diagnosed, a commercial laboratory can perform a tissue test for around $50. If a cheaper diagnosis is desired, sweet corn can be planted as a bioindicator. This plant expresses the deficiency symptoms very clearly and unambiguously if there is something lacking.
Correcting nutrient deficiencies
Having identified a nutrient problem, consider first whether there is some management problem that can be corrected first. For example, yellow leaves can be a symptom of both over and under-watering, as well as nitrogen or sulfur deficiency. Iron chlorosis is associated with water-logged conditions, and until irrigation is corrected, iron chlorosis will persist no matter how much iron chelate is applied. Also, subtropicals, such as lemon and avocado will often have yellowish leaves in cooler parts of the year, and will naturally green up when the weather turns warmer. The long term correction may not be additions of fertilizers, but selection of the appropriate plant for the conditions or the correction of the soil pH.
A fertilizer by law must have a guaranteed analysis. Synthetic fertilizers, such as urea or ammonium nitrate can easily fulfill this requirement. On the fertilizer container are the nitrogen (N): phosphorus (P): potassium (K) ratio. The numbers on the container, like 15:15:20, indicate the percentage of each of the three nutrients in the fertilizer. Due to the nature of the method of analysis, the phosphorus percentage is actually the percentage of phosphate (P2O5) and the potassium is actually potash (K2O). To correct for the percentage as their elemental forms, multiply them respectively by 0.43 and 0.83.
Synthetic fertilizers are characteristically fast acting, generally low in cost per pound of actual nutrient and some acidify the soil. Their disadvantages are that being salts, if they are mismanaged, they have a burn potential and if a nitrogen fertilizer, the potential for leaching of nitrogen is high. Slow-release nitrogen fertilizers are available at much higher cost and their release rates are governed by such environmental factors as soil moisture content and temperature.
A fertilizer is termed "complete" if it contains N,P and K; and "incomplete," if it is missing one or more of these. The term "complete" should not be interpreted as meaning that these are the only nutrients a plant needs. In fact the container may have other constituents than those listed on the label, but since their proportions cannot be consistently guaranteed, they are not listed. This is the case with manures like cow and chicken. Since their analysis varies from season to season and for the length of time they have been exposed to decomposition, it is difficult to guarantee the analysis. For this reason manures are not even legally fertilizers, although they are more complete than most fertilizers since they contain most nutrients required to grow plants. Organic concentrates, such as bonemeal, cottonseed and fish emulsion, also contain a variety of nutrients, but their cost per pound of nutrients is high relative to synthetic fertilizers.
The principal limitations to organic amendments is their bulk, availability, odor, potential salt and weed seed hazards, and expense per pound of actual nutrient. However, the value of manures and amendments does not lie solely in their nutritional additions, but also on the physical and biological effects they have on soil and plants. Organic amendments decrease soil bulk density, improve infiltration and water and nutrient holding capacities, and often increase disease resistance in plants.
Suggested Fertilizer Rates for Home Gardens
Table 3 shows the approximate nutrient content of manures, the analysis of a few representative fertilizers, and suggested yearly rates of application per 1,000 square feet of garden area. (The rates given are for materials used singly. If combinations of two or more materials are used , reduce the rates accordingly.) Table 4 gives suggested amounts of a few commercial fertilizers per 100 feet of row when rows are spaced 1, 2, 3 feet apart. (The number of measuring cups given is approximate, based on the density of the fertilizer.) The amount of material applied by a combination of broadcast and sidedress should not exceed the total amount for the material shown in Table 3.
Table 3. | ||||
Type of Manure
or Fertilizer |
Nitrogen
(N) |
Phosphorus
(P2O5) |
Potassium
(k2O) |
Suggested
Amounts of Material (pounds) |
chicken manure, dry
|
2-4.5
|
4.6-6.0
|
1.2-2.4
|
125
|
steer manure, dry
|
1-2.5
|
0.9-1.6
|
2.4-3.6
|
450
|
dairy manure, dry
|
0.6-2.1
|
0-1.1
|
2.4-3.6
|
600
|
calcium nitrate
|
15.5
|
0
|
0
|
16-25
|
ammonium nitrate
|
33.5
|
0
|
0
|
7-12
|
Ammonium sulfate
|
21
|
0
|
0
|
12-19
|
urea
|
46
|
0
|
0
|
12-19
|
19-9-0
|
19
|
9
|
0
|
13-21
|
16-20-0
|
16
|
20
|
0
|
16-25
|
12-12-12
|
12
|
12
|
12
|
12
|
Table 4. | ||||||
Type of Fertilizer
|
Row Spacings*
|
|||||
1 foot
|
2 feet
|
3 feet
|
||||
pounds
|
cups
|
pounds
|
cups
|
pounds
|
cups
|
|
calcium nitrate
|
1
|
1.4
|
2
|
2.8
|
3
|
4.2
|
Ammonium
|
.75
|
1.5
|
1.5
|
3
|
2.25
|
4.5
|
ammonium nitrate
|
.45
|
1
|
.9
|
2
|
1.35
|
3
|
urea
|
.35
|
1
|
.7
|
2
|
1
|
3
|
superphosphate
|
.5
|
1
|
1
|
2
|
1.5
|
3
|
treble
|
.25
|
.5
|
.5
|
1
|
.75
|
1.5
|
12-12-12
|
1.25
|
2.5
|
2.5
|
5
|
3.75
|
7.5
|
*Materials to be applied by sidedressing per 100 feet of row length for the row spacing indicated.
|
Soil Moisture Retention and Availability
Certain terms have become associated with the degree of soil saturation. Field capacity describes the amount of water a soil holds when it has drained of free water. Because a clay soil has more particles in it for a given weight than sandy soils, it will have more surface area and tend to have a larger surface attraction for water. The clay soil also has more pores than a sand soil. It will therefore tend to hold more water than a sand. If a plant is present and is removing water from the soil, the plant will transpire until water in the unsaturated condition moves so slowly that the plant will wilt. If the plant cannot recover, it is permanently wilted. A soil in this condition is said to be at the permanent wilting point (PWP). There may still be water present in the soil, but it is not available to the plant. The water in the soil that is present between field capacity and permanent wilting point is called available water, water that a plant can remove relatively easily.
Generally speaking, as the clay content of the soil increases, the total amount of water that the soil will hold also increases. Why? Because the total porosity increases. But not all of that increased water held by the soil is available. Table 5 shows how available water changes with soil texture. This will vary tremendously according to clay content, clay type and organic matter. For example, sand at field capacity can vary from 4 - 8% (g H2O/100g soil) water by soil weight and a clay loam can vary from 25 - 35%. And additions of organic matter can raise water holding capacity, as well as nutrient holding capacity immensely depending on the soil's content.
Table 5. Soil moisture characteristics of various soil textures. | |||
Soil Texture
|
Permanent Wilting Point
(gH2O/100g soil) |
Field Capacity
(gH2O/100g soil) |
Available Water
(gH2O/100g soil) |
Fine sand
|
1.7
|
6.8
|
5.1
|
Loam
|
6.8
|
18.1
|
11.3
|
Silt loam
|
7.9
|
19.8
|
11.9
|
Clay loam
|
10.2
|
26.5
|
16.3
|
Clay
|
14.7
|
28.6
|
13.9
|
The trends in the table demonstrate the increase in field capacity with clay content. But along with increased moisture content at field capacity, there is a greater amount of water held at permanent wilting point (Figure 3). Why? The increased surface tension that comes with a finer texture. See how a clay loam actually might have more available water than a clay, because the water content at PWP for the clay is much greater than that of the clay loam.
Figure3. Available water changes with soil texture.
Scheduling Watering
Adequate scheduling of watering in the home garden is essential for good plant growth. Applying too little or too much water is the main reason that most gardens fail to produce quality plantings. It is very difficult to give specific advice on the irrigation of all gardens. Landscapes that are put on a irrigation controller, frequently over or under irrigate depending on the season.
There are many factors which determine when to water your garden. Some factors are soil texture, age/size of plants, rooting depth, time of year, and whether or not there is a mulch-covering. An important idea to remember is that water is the number one limiting nutrient for plants in most of California. For a given increment of water up to a point, there is growth increase. This growth increase might be highly desirable if growing a food crop like corn or apples, but undesirable for a shrub that is just going to require more pruning. A little bit of stress can be beneficial in most landscapes. Since not all plants can sustain the same level of water stress, it is advisable to put plants of a similar drought tolerance together.
One method of scheduling involves simply allowing a slight wilting to occur to the plant before irrigating. This can be a bit like Russian roulette, since it requires paying attention and being present to water as soon as given level of stress is noted. Allowing a plant to stress for too long, predisposes it to diseases, such as various root rots. One idea to keep in mind is that different types of plants can support more stress than others, and they should be grouped accordingly in the landscape.
Another method of scheduling is by soil moisture content. This entails taking a soil sample from the root zone. The soil surface dries quite rapidly and is not representative for mature plants. Take a trowel or shovel and dig down six to eight inches for most established plants. A rule of thumb for timing irrigations according to moisture content is to water at 50% depletion of available water. A soil that has about 50% of its available water remaining will feel as follows:
Texture
Coarse - appears almost dry, will form a ball that does not hold shape
Loamy - forms a ball, somewhat plastic, will form weak ribbon, dark color
Clayey - forms a good ball, makes good ribbon, dark color, slightly sticky.
A third timing method involves the use of portable soil moisture meters. There are some inexpensive meters on the market ($10-15), which are robust enough to handle being inserted into the soil. These could be used exclusively to schedule, or used to train a person to the soil moisture or color that indicates 50% available water. After learning the cues, the individual would not need the meter.
Consideration of the watering system used - sprinkler, drip or furrow - is important. There are so many devices one can buy these days, it can be quite confusing. And everyone knows that drip is the most efficient, right. Well, any of these methods can apply water efficiently. In fact the most efficient method for trees or shrubs, is to create a small basin around the plant. A second berm of earth is built several inches away from the trunk to protect the trunk and crown roots from being water logged. The basin is rapidly filled with a two-inch depth of water. Other methods can be efficient, but proper design and then maintenance are the keys to an efficient system. Drip or other microsprinkler systems are no better than their management. On hilly, uneven terrain, a drip system might be the answer, but if the emitters clog from algae, sand or debris, then the system is not doing its job.
In deciding how long to irrigate, there are essentially two methods depending on whether one sprinkle irrigates or drip, furrow or basin irrigates. With all the methods one can run the system for a specified amount of time, then dig down to see the depth that water has infiltrated. In most cases water should infilter to the rooting depth of the plant or about 2 - 3 feet in depth for mature shrubs and trees. A piece of steel bar can also be inserted into the ground. The bar can be inserted easily to the depth of wetting, at which point it will become more difficult to go further. With basin and furrows, fill them with water for about 30 minutes one day, then the next day check for the depth of infiltration. Waiting is important because the water continues to drain through the soil long after the water has been turned off. If the depth of application has been exceeded or not obtained, run the system for a shorter or longer time.
With sprinklers, another method can be used to determine the length of time for an irrigation. Small cans can be spread throughout the garden and they will catch the amount of water applied with the irrigation. For most soils, sprinkling until two to three inches has accumulated in the cans will give a sufficient irrigation for mature shrubs and trees. This may take a number of hours. If the water intake of the soil is less than the application rate of the sprinklers, run-off will occur. Since run-off is not desired, either run the system intermittently, turning the water off and allowing infiltration to occur before resuming the irrigation, or change to smaller size sprinkler nozzles.
REFERENCES
UC Leaflets
- 2258-Soil and water management for home gardeners
- 2572-Organic and inorganic fertilizers and soil amendments
- 2637-What on ear