- Author: Ben Faber
Senior Public Information Officer
- UC Riverside,Plant materials that would otherwise become trash may be the key to solving two big problems: diminishing freshwater supplies for farms and diminishing effectiveness of antibiotics.
On average, agriculture accounts for 70% of global freshwater use. In California, which produces nearly half of all U.S.-grown fruits, nuts, and vegetables, that number rises to 80%.
The United Nations estimates food production will need to double by 2050. However, water supplies will not increase accordingly. Instead, due to climate change and drought, water resources are quickly shrinking.
One solution to the increasing need for farm water is to use treated municipal wastewater. There are roughly 16,000 wastewater treatment plants in the U.S., each of them capable of processing up to 10 million gallons every day.
“It's a huge amount of processed water that's mostly clean and can be used again, but there's a problem,” said Ananda Bhattacharjee, assistant project scientist at the U.S. Department of Agriculture's Salinity Laboratory, based at UC Riverside.
“This water can contain chemicals of emerging concern, like antibiotics, that are difficult to detect and treat without advanced and expensive instrumentation,” he said. “These instruments also require trained laboratory personnel to operate and maintain.”
Once exposed to the antibiotics in the water supply, soil bacteria immediately start developing resistance to the drugs because they want to survive. “Bacteria are amazing biological sensors,” he said. As the bacteria develop resistance, antibiotics stop working.
Once crops are irrigated with contaminated reclaimed water, plants that get harvested and come to our dinner tables may contain residual antibiotics, resistance genes, and resistant bacteria.
To correct this issue, Bhattacharjee is leading a new, $1 million project testing a low-cost technology to make the reclaimed water safer for agricultural re-use. Funded by the USDA's Agriculture and Food Research Initiative, the project will test how effectively biochar made from various types of discarded plant materials can “polish” the water.
Biochar is a charcoal-like substance made by burning organic material. Burning any organic matter, even wood chips, in limited-oxygen environments retains the mass of the burned substance. The remaining, charred substance is highly absorbent.
“It's like activated charcoal used in HEPA filters and HVAC systems. Biochar works on the same principal; it adsorbs chemicals present in reclaimed water and allows only clean water to pass through,” Bhattacharjee said.
Based on this principle, Daniel Ashworth, a soil scientist at the Salinity Laboratory, first built a bench-scale filtration system with biochar for the removal of antibiotics in synthetic wastewater. The results were very promising, with antibiotics removal efficiency of up to 98%.
“Encouraged by Dr. Ashworth's experiments, we will be designing the larger-scale biochar-based polishing systems for removing residual antibiotics in reclaimed water,” Bhattacharjee said.
Using biochar polishers could potentially remove the need to detect the antibiotics in reclaimed water, assisting treatment plants that do not have advanced detection or treatment technologies, and cannot afford them.
Affordability is one of the best features of the biochar system. “As engineers, we try to keep it simple. If we can build something for a dime, we don't want to have to spend a dollar,” Bhattacharjee said.
For this project, scientists from UC Riverside, the U.S. Department of Agriculture, US Salinity Laboratory, and the University of California's Agriculture and Natural Resources are teaming up to test biochar made from multiple kinds of plant materials left over from agricultural field production.
To start, they'll collect treated sewage sludge and plant materials such as pistachio shells and date palm leaves which would otherwise be thrown away. These materials will be turned into biochar for designing filtration systems that reclaimed water can pass through.
Ultimately, the team would like to develop a database of different, inexpensive biochar materials that can all be used for removing harmful compounds from reclaimed water for agricultural reuse, especially crop irrigation.
If the costs remain low and effectiveness remains high, the research team hopes growers will install biochar-based reclaimed water polishing systems on their farms. “That is the major goal of the project, taking this from bench scale to full field scale,” Bhattacharjee said.
Right now, the whole ecology of fields is changing due to residual antibiotics in irrigation systems. The reclaimed water gets into the soil, earthworms feed on organic matter in the soil, and they develop antibiotic resistance in their guts. Then they may release this resistance through their feces, making additional changes to soil microflora, which keeps the cycle of resistance going.
“We are slowly spiking our own agricultural fields with this resistance,” Bhattacharjee said. “Demonstrating this issue was our first project, Bacteria Wars: episode one. Now we have a technique to remove the antibiotics and resistant bacteria, reducing the antimicrobial resistance spread in agriculture. This is our episode two: Researchers Strike Back.”
subscribe to the Topics in Subtropics blog: https://ucanr.edu/blogs/blogcore/subscribe.cfm
- Author: Ben Faber
The Department of Water Resources (DWR) developed the Underrepresented Community Technical Assistance Program (URC TA Program) after learning that some Groundwater Sustainability Agencies (GSAs) have not been able to address the needs, risks, and vulnerabilities with the implementation of the Sustainable Groundwater Management Act (SGMA) to a sufficient extent within their Groundwater Sustainability Plan(s) (GSPs) or Alternative to a GSP (Alternative). DWR recognizes the need for addition assistance and, thus, implemented the URC TA Program to address this need and is the sole mission of the URC TA Program.
The mission of the URC TA Program is to determine the needs, risks, and vulnerabilities with the implementation of the Sustainable Groundwater Management Act (SGMA) for URCs in medium and high priority basins, including critically overdrafted (COD) basins.
DWR retained the services of a consultant group in 2021 to begin the URC TA Program utilizing Proposition 68 funds.
DWR is assisting Tribes and other URCs identified in the heat Maps found below. The URC TA Program will provide onsite engineering, geologic, hydrologic, and other technical services to the communities based upon a ranking of water systems. The types of services provided include, but are not limited to:
- Groundwater level monitoring
- Aquifer testing to determine long-term yield and supply
- Identifying Groundwater Dependent Ecosystems (GDEs)
- Analyze well interference
- Identifying additional water supply
- Analyze existing well condition using downhole video log
- Rehabilitation of water storage tank
- Long-term water supply and demand analysis
- Analyze and help to facilitate water transfers
In addition, DWR hired a nongovernmental agency (NGO) in 2022, using General Funds provided by the Budget Act of 2021, to expand upon the URC TA Program. The additional services DWR is providing through this agreement will:
- Create a template for local domestic well impact mitigation to develop plans for drinking water well protection. Share the data with three (3) local GSAs for the GSAs to develop a mitigation program. Provide ongoing community engagement by attending the three GSAs board meetings or committee meetings to submit the community's needs, risks, and vulnerabilities of URCs in their basin to amend GSPs or Alternatives.
- Analyze and provide feedback on proposed policies and programs by submitting written feedback to the three identified GSAs a minimum of three times a year for two years.
- Revise the existing Communication and Engagement Plan (CEP), if needed.
- Develop educational and outreach materials, if needed.
- Work with the three GSAs and community leaders previously identified to support and implement drinking water and wastewater service projects to help reduce vulnerabilities to groundwater contamination by providing written feedback to those three GSAs.
- Develop a financial strategy plan for the three GSAs to identify long-term strategies for addressing impacts of depleting groundwater supplies, drought, and climate change.
- Identify two common groundwater dependent vegetation species and develop groundwater thresholds that would lead to irreversible transition from a stable state of ecosystem structure. Develop a technical memo with the results of the study and datasets to support incorporation of results into GSP updates.
DWR was provided an additional $9.5 million in General Funds to expand the definition of a URC to include small and socially disadvantaged farmers and to provide technical assistance to the small farmers located within medium and high priority basins and COD basins. Additional information will be provided in early 2023.
Local entities will be able to request services through the SGM_TA@water.ca.gov email address managed by SGM Grant Program Team.
The Technical Assistance Program identifies communities throughout California with water supply challenges such as dry groundwater wells, water shortages, or poor water quality. Using publicly available data, DWR prepared a community identification tool (CIT) that collects relevant information for each census block within the State of California. The data includes, but is not limited to, Median Household Income (MHI), threats to groundwater, access to drinking water, and surface water quality. This information is used to quantify areas of greatest water-related need and applied statewide for Tribal Communities and within hydrologic regions including Northern California, Central California, and California's Central Coast for other Underrepresented Communities. For more information on the Underrepresented Community Prioritization Tool methodology, please refer to the Technical Memorandum Summary.
https://water.ca.gov/Work-With-Us/Grants-And-Loans/Sustainable-Groundwater/Underrepresented-Communities-Grants
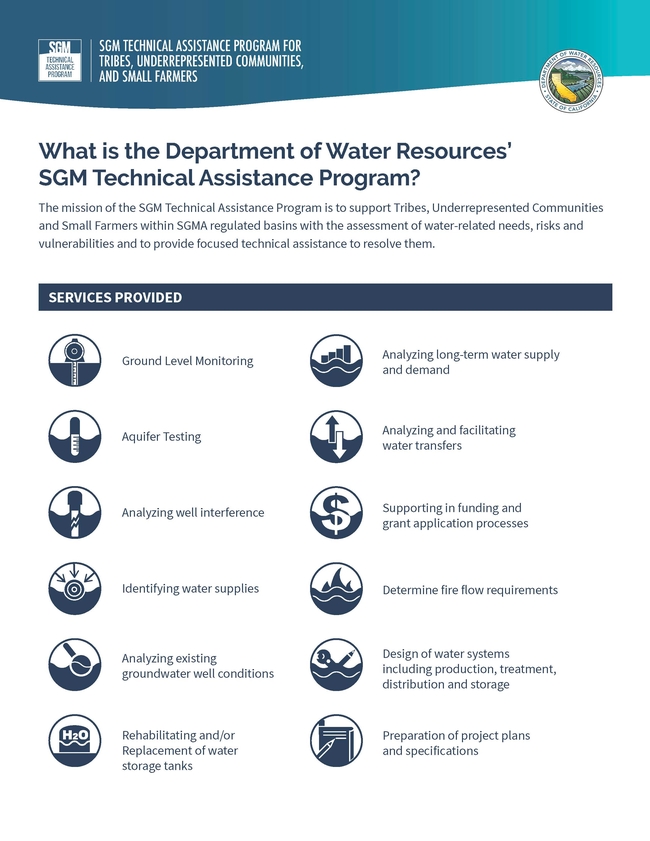
- Author: Pam Kan-Rice
To achieve groundwater sustainability under California's Sustainable Groundwater Management Act, or SGMA, demand management – policies that encourage water conservation – will be necessary, says Ellen Bruno, University of California Cooperative Extension specialist in quantitative policy analysis at UC Berkeley.
A key feature of the state's approach to SGMA is that local groundwater sustainability agencies can develop their own plans for achieving sustainable groundwater use, allowing for local flexibility and experimentation.
Reflecting the open-ended nature of the law, Groundwater Sustainability Plans across the state include a variety of water conservation strategies. Many, but not all, GSPs include tools such as allocations, taxes or fees, pumping restrictions, or efficiency incentives. Understanding these policy choices is important because they will influence the economic costs of SGMA.
To help people understand groundwater sustainability plans for their area, Bruno and UC Berkeley Ph.D. student Arthur R. Wardle have created a database. They record and explain the demand management proposals made in the state's 118 submitted groundwater sustainability plans and make these data publicly available in a new online platform called the SGMA Demand Management Action Database (SGMA-DMAD.com).
“The site allows for bulk download of the data we collected,” Wardle said. “Users can also locate a specific groundwater sustainability agency on a map of California to see what demand management strategies that agency is proposing.”
The Demand Management Action Database is the first easily navigated collection of the demand management strategies being proposed across California.
Many GSPs include over 1,000 pages, reflecting the many criteria they are required to satisfy. Among these pages are discussions of hydrogeologic features of the relevant groundwater subbasin, projections of future water demands and supplies, water budgets and other information necessary for the development of an effective management plan. Only one small section of the GSP, usually taking up only about a dozen pages, explains the management actions the agency is proposing to achieve sustainability.
“GSPs are an imperfect guide to what will actually happen as groundwater agencies implement SGMA,” Bruno said. “The plans laid out in GSPs are subject to change. However, submitted GSPs are the best publicly available evidence of what steps groundwater sustainability agencies plan to take in meeting their SGMA obligations.”
GSPs often distinguish between plans that will definitely be undertaken and plans that are provisional, subject to external approvals, need for additional funding, or are simply being left in the planning stage due to uncertainty over future water needs. The Demand Management Action Database includes information distinguishing between plans that are or will be implemented, versus those that remain uncertain.
“Some plans are still undergoing review and approval from the state,” Bruno said. “But even for the plans that have been approved, they have until 2040 to actually achieve their sustainability goals – or 2042 for basins not in critical overdraft – so I'm sure there will be adjustments to these proposals over time.”
In evaluating the role of demand management in submitted GSPs, the authors categorized demand management actions into a few broad categories – allocations, taxes/fees, pumping restrictions and efficiency incentives – each with a good deal of variation within them.
For each GSP, the Demand Management Action Database shows whether some policy fitting into each of these buckets is discussed in the GSP, along with details and page numbers for people wanting to know more about how a specific GSP is implementing each policy.
For those wanting to conduct their own analysis, the site also includes a download button enabling the entire database to be accessed at once. This data is free to use with proper citation.
Anyone interested in SGMA governance is invited to explore the site and send questions, comments or concerns to Arthur R. Wardle at arw@berkeley.edu.

- Author: Ben Faber
Thelma Hansen Symposium to Explore the Future of Water in Agriculture
Webinar Series
Presented by The Hansen Agricultural Research & Extension Center
Three Consecutive Days of Discourse
Each webinar will open to participants 5 minutes before 4:00 PM.
See the Full Schedule: https://ucanr.edu/sites/PSU/files/383979.pdf
Tuesday, May 23: Water Availability
4:00 PMWelcome—Annemiek Schilder, PhD, Director UCCE/HAREC Ventura County
4:05Opening remarks—Kelly Long, Supervisor Ventura County District 3
4:15Long-term forecasting of trends in California water management—Erik Porse, PhD, Director,
UC ANR, California Institute for Water Resources
4:50Groundwater level changes and water well drilling along California's Central Coast and
around the globe—Scott Jasechko, PhD, Bren School of Environmental Science &
Management, UC Santa Barbara
5:30Closing—Annemiek Schilder
Wednesday, May 24: Water Quality
4:00 PMWelcome—Annemiek Schilder, Director UCCE/HAREC Ventura County
4:05Focus on regional water quality—Norma Camacho, Chair, Los Angeles Regional Water
Quality Control Board
4:20Nitrate, pesticides, and sustainable groundwater quality management in agricultural
landscapes—Thomas Harter, PhD, Department of Land, Air and Water Resources,UC Davis
5:05New regulations affect water quality management in Ventura County—Jodi Switzer, Ventura
County Agricultural Irrigated Lands Group
5:30Closing—Annemiek Schilder
Thursday, May 25: Water Economics
4:00 PMWelcome—Annemiek Schilder, Director UCCE/HAREC, Ventura County
4:05Economics of water in California agriculture—Richard Howitt, PhD, Emeritus Professor,
Center for Watershed Sciences, UC Davis
4:50Water Markets: the importance of good design—Matthew Fienup, PhD, Executive Director,
Center for Economic Research & Forecasting, California Lutheran University
5:30Closing—Annemiek Schilder
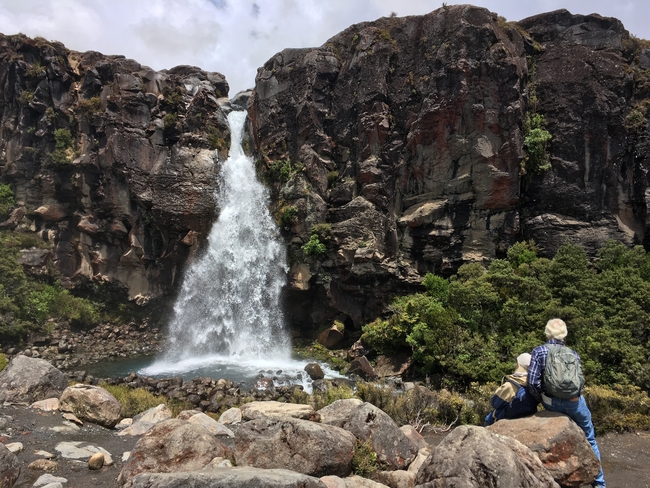
- Author: Craig Kallsen
To maximize profits in the early navel orange market, growers need to have large fruit size and sufficient yellow-orange color and a high enough sugar-acid ratio to meet or exceed the legal minimum harvesting standards. Growers of early-maturing navel oranges in Kern County use different strategies to produce these oranges. Some growers irrigate at full evapotranspiration rates nearly up to harvest with the belief this will maximize fruit size, while others begin deficit irrigating a month or two prior to harvest to maximize development of sugar and color to promote earlier maturity. Little information exists in the literature to assist growers in making decisions related to producing early maturing navels such as Beck, Fukumoto and Thompson Improved. To determine the effects of late season irrigation stress, I, along with two University of California co-researchers Blake Sanden and Dr. Mary Lu Arpaia, participated in an experiment to elucidate some of the trade-offs that relate to irrigation strategies and early navel fruit production. The research was conducted from 2006 through 2008 in a cooperating grower's Beck orchard at the extreme southern end of the San Joaquin Valley. Our generous and patient cooperating growers were George and Colby Fry.
Three different irrigation treatments, defined as low, mid and high, were developed based on the relative amounts of irrigation water applied to the test plots. Each plot consisted of 10 trees in a central row, bordered by ten similarly irrigated trees in the two adjacent rows. Each treatment was replicated five times. The same irrigation treatment was applied to the same plots for the first two years, while in the third year the low treatment was changed to the high treatment to provide information on how rapidly the trees would recover from stress. The different irrigation treatments were administered by using irrigation emitters with different flow rates and by differentially shutting off water to some treatments as needed to achieve desired stress levels. Between growing seasons, the top three feet of soil profile was refilled with water during the winter and differential irrigation began in early August. Measurable differences in tree shaded stem water potential among treatment usually were noted by early September. In the second year of the experiment (2007), the low and mid-irrigation treatments applied approximately 38 and 71 percent, respectively on average, of the water of the high treatment. Water potential measurements made mid-day on shaded, interior leaves demonstrated that good separation was achieved among the three differential treatments. In 2007, for example, shaded stem water potential measurement in early September were about -9, -12, and -18 bars for the high, mid and low irrigation treatments, respectively and at harvest in mid-October were -12, -18, -24, respectively. Neutron probe measurements also demonstrated that trees differentially depleted available water stored in the soil as the season progressed (data not shown). In 2007, differences in applied water among the treatments were large. Including the increased quantity of water applied to refill the soil profile in the winter, 3.55, 2.58 and 2.11 acre feet of water on a per acre basis, were applied to the high, mid and low irrigation treatments respectively, from October 30, 2006, to harvest, October 15, 2007. Rainfall was minimal.
Again, using 2007 as an example, as the level of applied water decreased, soluble solids (i.e. sugars) and titratable acid, were greater at harvest, although the sugar acid ratio was not different (see Table 1).
Rows in the experimental orchard were oriented east and west. Fruit on the south side of the tree had higher soluble solids concentration and sugar/acid ratio than fruit on the north side of the tree, regardless of irrigation treatment. Fruit juiciness, either measured as weight of juice to weight of fruit (see Table 1) or volume of juice per weight of fruit (results not shown) were not different among irrigation treatments, suggesting the increase in sugars and acid was the result of osmotic adjustment and not fruit dehydration. We were also interested in seeing if the differential irrigation treatments influenced eating quality of the fruit. To test this idea, we provided fruit from the highest and lowest irrigation treatments of 2007 and 2008 to volunteer panelists at the UC Kearney Ag Center and asked if they could detect any differences between the fruit. Results from both years showed that the panelists could not detect differences between the two irrigation treatments. This suggests that the increase in soluble solids in the low irrigation treatment was not sufficient to influence eating quality.
In 2007, yield and grade decreased as the amount of applied water decreased (see Table 2).
Fruit in the high and mid irrigation treatments peaked on size 56 per carton and on size 72 per carton in low treatment (data not shown). The decrease in fruit grade at pack-out appeared to be largely due to a more oblong shape. The negative yield, fruit size and grade effects measured in the low and mid treatments in 2007 were probably the cumulative result of deficit irrigation in Years 1 and 2 and not just Year 2 alone. Reduced rates of irrigation hastened development of fruit color compared to the high irrigation treatment (see Table 3) and this occurred every year.
The deleterious effects on yield, and grade on the trees in the low-irrigation treatments suggested that not much would be gained by continuing this level of stress for a third season in the same plots. In 2008, the low irrigation treatment was replaced by a high irrigation treatment and, at harvest, yield by weight and fruit numbers were not different from the control high-irrigation treatment. This observation demonstrated that the Beck navels rebounded quickly from the low irrigation stress of 2006 and 2007. The mid-level irrigation stress of 2006 and 2008 was less severe than that of 2007, and yield and fruit quality was not as adversely affected as in 2007.
This study provides information on some of the trade-offs that might be expected among fruit yield, size, grade, sugar and color in relation to reduced irrigation as harvest approaches. More detailed information from the trial can be found at the following link: https://doi.org/10.21273/HORTSCI.46.8.1163. How growers respond to this information will depend on their approach to profiting in the early navel market and how much water will be available for irrigation. If reducing water use is the primary goal of the grower, while minimizing effects on yield and fruit quality compared to fully irrigated orchards, work by Dr. Goldhamer, UC irrigation specialist, demonstrated that regulated deficit irrigation in the mid-May through mid-July time period would be the best strategy. The authors gratefully acknowledge the Citrus Research Board for its financial support of this project.