Agrobiodiversity
Background
Managed agricultural soils differ from unmanaged (or ‘natural’) ecosystems in several key ways. While unmanaged systems tend to have high diversity of life forms as the norm, agricultural ecosystems (or agroecosystems for short) largely lack plant diversity as single crops dominate the landscape, which can depress diversity of other organisms such as microbes or mammals. [1, 2] Agroecosystems are fundamentally focused on exports of crop outputs and are therefore often dependent on large inputs of nutrients and water. On the other hand, natural systems are characterized by relatively low levels of inputs and exports.
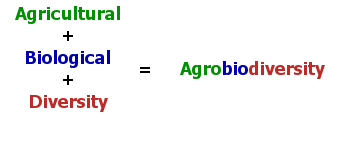
Agrobiodiversity and Nutrient Cycles
Nutrients in the soil exist in many forms and are constantly in a state of change. These patterns of changes and transformations are called ‘nutrient cycles.’ For example, nitrogen (N) can be part of large organic molecules such as proteins or partially degraded plant biomass. Soil microbes break down these larger molecules into smaller pieces, including the plant available ammonium (NH4+). This ammonium can then be transformed into nitrate (NO3-) by other soil microbes. Alternatively, microbes can take up N from the soil and use it to grow, returning N to organic forms that are no longer available to plants.

The activity of microbial communities in unmanaged environments can differ drastically from that in managed agricultural operations. One key difference is that nutrient cycles in natural environments tend to be more ‘closed’ than those in agricultural soils. Compared to an ‘open’ nutrient cycle, a ‘closed’ cycle receives fewer inputs while also losing less nutrients to the environment. For example, nitrate leaching and nitrous oxide losses are elevated in managed soils that receive large amounts of N fertilizers. Of course, maintaining harvestable yields (which represent a removal of nutrients from the system) requires some supplemental nutrient inputs. That being said, unmanaged systems are increasingly being examined to develop agricultural practices that limit nutrient losses by closing nutrient cycles and maximizing the use of the soil system’s inherent ability to provide nutrients. [6]
One recent study in an organic tomato operation in California showed that careful organic fertilizer management created such a tight N cycle that yields exceeded the average county yields. Despite significant yield decreases generally associated with organic farming, the average yield of the most efficient systems studied (42.8 tons/ac) exceeded the average county yield (38.5 tons/ac), which included mostly conventional operations. These yields were found in fields that had the highest levels of readily available C and N supplied through a diverse array of organic inputs, resulting in a high level of microbial activity. [7]
Crop Rotations
Rotating crops grown on a particular piece of land is a relatively straightforward way to maintain high levels of agrobiodiversity. A variety of crop rotation strategies exist, with the chosen approach depending on the desired goal.
Utilizing a leguminous crop such as alfalfa increases soil N and can minimize the fertilizer requirements for the following crop. A nitrogen credit between 60-130 lbs N/ac can be expected following alfalfa incorporation. [8] Alternatively, residual soil nitrogen can be managed by planting crops with high nitrogen uptake capabilities following crops that are fertilized at high rates, which can prevent losses of soil nitrogen via nitrate leaching. Rotating crops can also help break reproduction patterns of crop pests and soil-borne diseases while helping mitigate the proliferation of weeds.
Some popular crop rotations in California include tomato-wheat in the San Joaquin Valley and lettuce-lettuce-cole in the Salinas Valley.
Cover Crops
Cover cropping refers to the planting of crops that are grown for reasons other than providing a direct cash income. Cover crops that are planted to increase soil nitrogen are referred to as ‘green manures’ while cover crops planted to retain residual soil nitrogen are known as ‘catch crops.’ For more information about cover cropping visit our cover crops focus topic. See the section below on soil organic matter for more about the benefits of cover crops.
Intercropping
The practice of intercropping involves planting a variety of crops in close proximity to one another. [9]
There are four main types of intercropping:
1) Mixed Intercropping: Different crops planted together with no clear row patterns
2) Row intercropping: Alternating rows of varying crops
3) Strip Intercropping: Growing varying crops in strips
4) Relay Intercropping: Staggering planting to attain overlap in crop growth
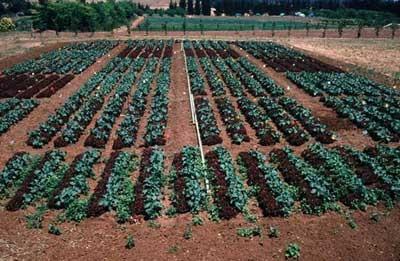
Alternatively, intercropping a plant that attracts organisms that prey on common crop pests can serve as an alternative to pesticide applications. In California, lettuce-alyssium intercropping is used in this way. The alyssium plants attract hoverflies whose larvae prey on currant-lettuce aphids. [11]
Intercropping cereals and legumes is another popular approach practiced in the United States, though this isn’t commonly done in California.
Soil Organic Matter
Converting soils to agricultural production results in significant losses of soil organic matter, in large part due to the breaking down of soil aggregates that comes with soil disturbance such as tillage. Practices that increase agrobiodiversity can be used to address this loss of soil organic matter.
Crops differ both in the amounts of residue that they add to the soil and also in the chemical makeup of those residues. Incorporating crops with higher levels of residue inputs into crop rotations can help protect against losses of soil organic matter. For example, incorporating a legume cover crop into a wheat system can accumulate soil organic matter instead of depleting it. [12] A recent analysis of 122 studies found that crop rotations increased total soil C by 3.6%. When those rotations included a legume, soil C was increased by 8.5%. Rotations also increased the total microbial biomass by over 20%. [13]
Sources
- Cleland, E.E. and W.S. Harpole, Nitrogen enrichment and plant communities, in Annals of the New York Academy of Sciences. 2010. p. 46-61.
- Clark, C.M., et al., Environmental and plant community determinants of species loss following nitrogen enrichment. Ecology Letters, 2007. 10(7): p. 596-607.
- Baas-Becking, L.G.M., Geobiologie of Inleiding Tot de Milieukund. 1934, The Hague: Van Stockkum & Zoon.
- Kamaa, M., et al., Effects of organic and inorganic fertilization on soil bacterial and fungal microbial diversity in the Kabete long-term trial, Kenya. Biology and Fertility of Soils, 2011. 47(3): p. 315-321.
- Malý, S., J. Královec, and D. Hampel, Effects of long-term mineral fertilization on microbial biomass, microbial activity, and the presence of r- and K-strategists in soil. Biology and Fertility of Soils, 2009. 45(7): p. 753-760.
- Gliessman, S.R., Agroecosystem Sustainability: Developing Practical Strategies. 2001, United States: CRC Press.
- Bowles, T.M., et al., Tightly-Coupled Plant-Soil Nitrogen Cycling: Comparison of Organic Farms across an Agricultural Landscape. PLoS ONE, 2015. 10(6): p. e0131888.
- Putnam, D.a.E.L., Characterizing N fertilizer requirements of crops following alfalfa. 2016, Fertilizer Research and Education Program Project Summary: University of California, Davis.
- Ofori, F. and W.R. Stern, Cereal–Legume Intercropping Systems, in Advances in Agronomy, N.C. Brady, Editor. 1987, Academic Press. p. 41-90.
- Broccoli/Lettuce Intercropping in California, USA. [cited 2016 9/20/2016]; Available from: http://www.agroecology.org/Case%20Studies/broccoli_intercrop.html.
- Brennan, E. Flower Power Protects Organic Lettuce Fields. 2014 9/20/2016]; Available from: https://agresearchmag.ars.usda.gov/2014/jan/lettuce/.
- Miglierina, A.M., et al., The effects of crop rotation and fertilization on wheat productivity in the Pampean semiarid region of Argentina. 1. Soil physical and chemical properties. Soil and Tillage Research, 2000. 53(2): p. 129-135.
- McDaniel, M.D., L.K. Tiemann, and A.S. Grandy, Does agricultural crop diversity enhance soil microbial biomass and organic matter dynamics? A meta-analysis. Ecological Applications, 2014. 24(3): p. 560-570.