- Author: Surendra K. Dara
Entomopathogenic fungi (EPF) are those that infect various arthropods such as ticks, mites, and insects. There are two major groups of EPF that play an important role in pest suppression. Members of the order Entomophthorales are more host-specific and examples include Entomophaga maimaiga in spongy moth, Entomophthora muscae and Strongwellsea spp. in flies, Conidiobolus obscurus, Entomophthora planchoniana, Neozygites fresenii and Pandora neoaphidis in aphids, and Neozygites floridana in mites. These naturally occurring EPF are fastidious and cannot be mass produced on a commercial scale, but cause epizootics when host populations are high and environmental conditions are favorable resulting in significant pest suppression. On the other hand, members of the order Hypocreales are more generalistic pathogens and can be infective to a variety of arthropods. Beauveria bassiana, Cordyceps fumosorosea, Hirsutella thompsonii, and Metarhizium brunneum are some examples of hypocrealeans. These can be grown on artificial media on a commercial scale and several biopesticide formulations based on various isolates of these fungi are available in the US and elsewhere. Both entomophthoralean and hypocrealean fungi have the same mode of infection. When fungal spores come in contact with their host, they germinate and enter the host body through mechanical pressure and enzymatic degradation of the cuticle. They multiply inside the host, invade the tissues, and finally emerge from the cuticle to produce spores that continue the infection process.
With growing emphasis on sustainable crop production with safer pesticides, the market for biopesticides including EPF-based ones has been increasing. Newer EPF isolates and modern technology contributed to the development of improved formulations. EPF-based products can be used for soil-inhabiting pests or their life stages like root aphids, pupae of thrips, and wireworms to foliar feeders or above-ground pests including the members of Coleoptera, Diptera, Hemiptera, Orthoptera, Thysanoptera, and others. Considering their potential against a variety of pests on multiple crops, EPF-based pesticides should be an important part of integrated pest management (IPM) programs. However, there is a significant knowledge gap in effectively using EPF in IPM and fully exploring their potential in sustainable crop production.
Since EPF spores need to come in contact with the host, using them against the right pest or life stage is very important to obtain desired results. Sometimes, using EPF in combination or rotation with botanical or synthetic pesticides is more effective than using them alone against a particular pest (Dara 2013; 2015; 2016). As EPF formulations contain live fungi, label instructions should be followed for proper storage, transportation, tank-mixing, and application to maintain their efficacy. Compatibility can vary according to the EPF and its formulation, but studies showed that some isolates of Beauveria bassiana and Metarhizium anisopliae are compatible with several fungicides (Dara, et al., 2014; Roberti et al., 2017; Khun et al., 2021).
In addition to controlling arthropod pests, EPF being soilborne fungi also have a direct relationship with plants and other microbes. EPF colonize plant tissues and grow inside the plants in a phenomenon known as endophytism. Endophytic EPF grow as hyphae and do not produce spores. Although they cannot cause infection to pests feeding on those plants, they indirectly affect pests by reducing their fitness and survival by activating induced systemic resistance. When EPF are applied to soil, they form a mycorrhiza-like relationship with plant roots and help plants withstand biotic stresses and improve nutrient uptake. EPF can also antagonize plant pathogens through competitive displacement and antimicrobial activity. Thus soil and foliar application of EPF-based pesticides result in additional benefits in improving crop growth and health in addition to controlling pests through infection.
Several studies explored the non-entomopathogenic roles of EPF (Dara, 2019a). Soil application of B. bassiana had a positive impact on the survival, growth, and health of cabbage plants growing under water stress (Dara et al., 2017). Metarhizium brunneum also had a similar impact on plant growth in this study. Root and rhizosphere colonization by Metarhizium spp.improved shoot length and root weight in industrial hemp (Hu et al., 2023) and root colonization of Metarhizium robertsii alleviated hemp from salt and drought stress. Metarhizium spp. and B. bassiana transferred nitrogen from dead insects to the plant they colonized (Behie et al., 2012; Behie and Bidochka, 2014). These studies show the role of EPF in soil nitrogen cycle and how plants benefit from the endophytic relationship of EPF. Additionally, recent reports showed that endophytic B. bassiana induced the biosynthesis of flavonoids in oilseed rape (Muola et al., 2023) and flavonol content in licorice plants (Etsassala et al., 2023).
Seed treatment with B. bassiana increased plant height, stem diameter, number of leaves, shoots and apical buds, biomass, and total chlorophyll content in cotton and reduced cotton aphid (Aphis gossypii) populations (Mantzoukas et al., 2023). Similarly, endophytic B. bassiana significantly reduced the reproductive rate and populations of the Russian wheat aphid (Diuraphis noxia) in South African wheat (Motholo et al., 2023). In corn, endophytic B. bassiana and M. anisopliae negatively impacted the survival, development, and reproduction of the fall armyworm (Spodoptera frugiperda) (Altaf et al., 2023).
Soil application of B. bassiana, Cordyceps fumosorosea, and Metarhizium brunneum antagonized Fusarium oxysporum f.sp. vasinfectum in cotton as effectively as some biofungicides (Dara et al., 2020). Beauveria bassiana treatment at a higher rate provided significantly better protection than all other treatments in this study. Both B. bassiana and C. fumosorosea inhibited the growth of F. oxysporum in vitro (Yanagawa et al., 2021). In corn, endophytic M. robertsii promoted plant growth and reduced southern corn leaf blight caused by Cochliobolus heterostrophus (Imtiaz et al., 2023). Induced systemic resistance is thought to be responsible for this protection. Similarly, B. bassiana applied as seed treatment, seedling root dip, and foliar spray reduced the incidence of rice sheath blight caused by Rhizoctonia solani by 69% and its severity by 60% under field conditions (Deb et al., 2023). Beauveria bassiana also resulted in 71% of mycelial inhibition in R. solani through the production of cell wall degrading enzymes, release of secondary metabolites, and mycoparasitism.
Multiple recent studies showed that EPF also have a negative impact on plant-parasitic nematodes. Beauveria bassiana and C. fumosorosea reduced the survival ofthe root-knot nematode, Meloidogyne incognita, in vitro (Yanagawa et al., 2021). Similar to the nematophagous fungus Purpureocillium lilacinum, both B. bassiana and M. anisopliae were effective in reducing galls caused by M. incognita in tomato and cucumber (Karabörklü et al., 2022). Metarhizium anisopliae was as effective as P. lilacinum with 75% reduction in gall formation and 85% control of second instar juveniles in tomato. Beauveria bassiana and M. anisopliae also resulted in about 85% control of second instar juveniles in cucumber. In another study, soil application of B. bassiana significantly reduced nematode infestation in tomato roots and B. bassiana treatment caused 60% mortality in nematodes in a lab assay (Kim et al., 2023). Volatile organic compounds, 1-octen-3-ol and 3-octanone from M. brunneum attracted and killed another plant-parasitic nematode, Meloidogyne hapla, in lab assays (Khoja et al., 2021).
As many of these recent studies indicated, the non-entomopathogenic roles of EPF is a new area of applied research interest with tremendous practical benefits. In addition to direct pest control through infection, EPF as endophytes offer multiple benefits in suppressing pest populations by affecting their fitness, antagonizing plant pathogens and plant-parasitic nematodes, imparting drought and salt tolerance in plants, improving nutrient uptake, and promoting overall growth and health of plants. Using EPF-based biopesticides comes under the microbial control of IPM (Dara, 2019b) and will contribute to insecticide resistance management. Additionally, the non-target benefits of EPF will help growers optimize the use of other inputs and related costs. EPF can be very important in sustainable crop production and a thorough understanding of their biology, interactions with pests, plants, pathogens, and other biotic and abiotic factors, and effective use strategies will help achieve their full potential.
Note: This article was initially published in the December 2023 issue of CAPCA Adviser magazine.
References
Altaf, N., M. I. Ullah, M. Afzal, M. Arshad, S. Ali, M. Rizwan, L. A. Al-Shuraym, S. S. Alhelaify, and S. Sayed. 2023. Endophytic colonization by Beauveria bassiana and Metarhizium anisopliae in maize plants affects the fitness of Spodoptera frugiperda (Lepidoptera: Noctuidae). Microorganisms 11: 1067.
Behie, S. W. and M. J. Bidochka. 2014. Ubiquity of insect-derived nitrogen transfer to plants by endophytic insect-pathogenic fungi: an additional branch of the soil nitrogen cycle. Appl. Environ. Microbiol. 80: 1553-1560.
Behie, S. W., P. M. Zelisko, and M. J. Bidochka. 2012. Endophytic insect-parasitic fungi translocate nitrogen directly from insects to plants. Science 336: 1576-1577.
Dara, S. 2013. Microbial control as an important component of strawberry IPM. CAPCA Adviser, 16 (1): 29-32.
Dara, S. K. 2015. Root aphids and their management in organic celery. CAPCA Adviser 18 (5): 65-70.
Dara, S. K. 2016. IPM solutions for insect pests in California strawberries: efficacy of botanical, chemical, mechanical, and microbial options. CAPCA Adviser 19 (2): 40-46.
Dara, S. K. 2019a. Non-entomopathogenic roles of entomopathogenic fungi in promoting plant health and growth. Insects 10: 277.
Dara, S. K. 2019b. The new integrated pest management paradigm for the modern age. JIPM 10: 12.
Dara, S. K., S. S. Dara, and S.S.R. Dara. 2020. Managing Fusarium oxysporum f. sp. vasinfectum Race 4 with beneficial microorganisms including entomopathogenic fungi. Acta Horticulturae 1270: 111-116.
Dara, S. K., S.S.R. Dara, and S. S. Dara. 2017. Impact of entomopathogenic fungi on the growth, development, and health of cabbage growing under water stress. Am. J. Plant Sci. 8: 1224-1233.
Dara, S.S.R., S.S. Dara, A. Sahoo, H. Bellam, and S. K. Dara. 2014. Can entomopathogenic fungus Beauveria bassiana be used for pest management when fungicides are used for disease management? UCANR eJournal of Entomology and Biologicals October 23, 2014.
Deb, L., P. Dutta, M. K. Mandal and S. B. Singh. 2023. Antimicrobial traits of Beauveria bassiana against Rhizoctonia solani, the causal agent of sheath blight of rice under filed conditions. Plant Disease PDIS-04. https://doi.org/10.1094/PDIS-04-22-0806-RE.
Etsassala, N. G. E. R., N. Macuphe, I. Rhoda, F. Rautenbach and F. Nchu. 2023. An endophytic Beauveria bassiana (Hypocreales) strain enhances the flavonol contents of Helichrysum petiolare. In Sustainable Uses and Prospects of Medicinal Plants, eds. L. Kambizi and C Bvenura, CRC Press. pp 367-377.
Hu, S. and M. J. Bidochka. 2023. Colonization of hemp by Metarhizium and alleviation of salt and drought stress. 55th Annual meetings of the Society for Invertebrate Pathology, July 30-August 3, 2023, College Park, MD, pp. 56-57.
Hu, S., M. S. Mojahid, M. J. Bidochka. 2023. Root colonization of industrial hemp (Cannabis sativa L.) by the endophytic fungi Metarhizium and Pochonia improves growth. Industrial Crops and Products 198: 116716.
Imtiaz, A. M. M. Jiménez-Gasco, and M. E. Barbercheck. 2023. Endophytic Metarhizium robertsii suppresses the phytopathogen, Cochliobolus heterostrophus and modulates maize defenses. 55th Annual meetings of the Society for Invertebrate Pathology, July 30-August 3, 2023, College Park, MD, pp. 66-67.
Karabörklü, S., V. Aydinli and O. Dura. 2022. The potential of Beauveria bassiana and Metarhizium anisopliae in controlling the root-knot nematode Meloidogyne incognita in tomato and cucumber. J. Asia-Pacific Entomol. 25: 101846.
Khoja, S., K. M. Eltayef, I. Baxter, A. Myrta, J. C. Bull and T. Butt. 2021. Volatiles of the entomopathogenic fungus, Metarhizium brunneum, attract and kill plant parasitic nematodes. Biol. Con. 152: 104472.
Khun, K. K., G. J. Ash, M. M. Stevens, R. K. Huwer, B. A. Wilson. 2021. Compatibility of Metarhizium anisopliae and Beauveria bassiana with insecticides and fungicides used in macadamia production in Australia. Pest Manag. Sci. 77: 709-718.
Kim, K. J., S. E. Park, Y. Im, H. Yang and J. S. Kim. 2023. Drenching of Beauveria bassiana JEF-503 reduces the root knot nematode populations in soil. 55th Annual meetings of the Society for Invertebrate Pathology, July 30-August 3, 2023, College Park, MD, pp. 68.
Mantzoukas, S., V. Papantzikos, S. Katsogiannou, A. Papanikou, C. Koukidis, D. Servis, P. Eliopoulos, and G. Patakioutas. 2023. Biostimulant and bioinsecticidal effect of coating cotton seeds with endophytic Beauveria bassiana in semi-field conditions. Microorganisms 11: 2050.
Motholo, L. F., M. Booyse, J. L. Hatting, T. J. Tsilo, M. Lekhooa, and O. Thekisoe. 2023. Endophytic effect of the South African Beuaveria bassiana strain PPRI 7598 on the population growth and development of the Russian wheat aphid, Diuraphis noxia. Agriculture 13: 1060.
Moula, A., T. Birge, M. Helander, S. Mathew, V. Harazinova, K. Saikkonen, and B. Fuchs. 2023. Endophytic Beauveria bassiana induces biosynthesis of flavonoids in oilseed rape following both seed inoculation and natural colonization. Pest Manag. Sci. DOI 10.1002/ps.7672
Roberti, R. H. RIghini, A. Masetti, and S. Maini. 2017. Compatibility of Beauveria bassiana with fungicides in vitro and on zucchini plants infested with Trialeurodes vaporariorum. Biol. Con. 113: 39-44.
Yanagawa, A., N.P.R.A. Krishanti, A. Sugiyama, E. Chrysanti, S. K. Ragamustari, M. Kubo, C. Furumizu, S. Sawa, S. K. Dara, and M. Kobayashi. 2022. Control of Fusarium and nematodes by entomopathogenic fungi for organic production of Zingiber officinale. J. Natural Medicines, 76: 291-297.
The western flower thrips, Frankliniella occidentalis (Pergande) (Thysanoptera: Thripidae) is one of the major pests of lettuce in California. It has a wide host range including several vegetable, ornamental, and other cultivated or wild plants. Native to North America, the western flower thrips is also known as alfalfa thrips, California thrips, and maize thrips among others. This article provides a general overview of the pest, its biology, damage, and management.
Biology:
Eggs are small, oval, and inserted into plant tissue. Nymphs are slender and have four instars. The first two - larva I and II – feed on plant tissues while the latter two - prepupa and pupa – are non-feeding stages that are often found in the soil. Larvae are wingless and white initially and turn yellow or orange once they start feeding. Adults are small (< 2 mm), slender, and have two pairs of long, narrow wings with a fringe of hairs. The western flower thrips can occur in different color morphs such as yellow or orange, brown, and black.
Damage:
The western flower thrips prefers flowers, but also feeds on developing buds, fruits, and foliage. Larvae and adults rupture the leaf surface with their rasping mouthparts and feed on plant juices. Feeding damage results in silvery appearance of the leaf surface, which later turns brown. The presence of dark fecal specs indicates thrips occurrence. In lettuce, the western flower thrips transmits Tomato spotted wilt virus and is the sole vector of Impatiens necrotic spot virus. Only the larval stages acquire these tospoviruses and the adults transmit the viruses to other plants as they spread in the field.
Management:
Integrated pest management approach is critical for successful pest management. It involves regular monitoring, exploring the potential of multiple options including cultural and biological solutions, and proper timing and application of various strategies among others. The western flower thrips is one of the pests where insecticide resistance is a common problem. To reduce the risk of resistance development, it is necessary to explore the potential of multiple control options and rotate insecticides with different modes of action. This is essential to suppress pest populations to desired levels and also to maintain control efficacy of existing pesticides.
Cultural control – Remove weed and other hosts that harbor thrips or viruses. Sprinkler irrigation can help reduce thrips populations. Plow down lettuce crop residue to destroy surviving stages. In general, maintaining good plant health with optimal nutrition and irrigation practices helps plants withstand pest damage. Silicate products can improve the structural strength of plant tissues and reduce pest damage and/or populations. Several biostimulants or biological soil amendments can also help activate plant's natural defenses against pest infestations. Consider using them to improve overall plant health and yields, and to protect plants from biotic and abiotic stresses.
Biological control – Predators such lacewings (Chrysopa spp. and Chrysoperla spp.), minute pirate bugs (Orius spp. and Anthocoris spp.), predatory mites (Amblyseius swirski, Ablyseius andersoni, Neoseiulus cucumeris and Stratiolaelaps scimitus), and rove beetles (Dalotia coriaria) attack thrips. Conserve natural enemies with insectary plants and applying safer pesticides, and augment natural populations by releasing commercially reared species.
Microbial control – Entomopathogenic fungi such as Beauveria bassiana and Cordyceps (Isaria) fumosorosea, products based on bacteria such as Burkholderia rinojensis and Chromobacterium subtsugae, and entomopathogenic nematodes such as Heterorhabditis spp. and Steinernema feltiae can be used against one or more life stages. Entomopathogenic nematodes are more effective against pupae in soil because they actively search for and infect their hosts. Entomopathogenic fungi can be used against all life stages.
Botanical control – Azadirachtin alone or in combination with entomopathogenic fungi or insecticides can also be used against multiple life stages. Azadirachtin is an insecticide, antifeedant, and a growth regulator. Similarly, pyrethrins derived from chrysanthemum flowers can be used alone or with other biological or synthetic insecticides. Pyrethrins are nerve poisons. Other botanical insecticides that contain soybean oil, rosemary oil, thymol, and neem oil (which also has a low concentration of azadirachtin) also provide control against thrips through insecticidal, repellency, and antifeedant activities.
Other control options – Insecticidal soaps and mineral oils can be used against different life stages of thrips. Spinosad, a popular insecticide of microbial origin and a mixture of two chemicals spinosyn A and spinosyn D, is very effective against thrips. However, overuse of spinosad can lead to resistance issues in thrips and other insects.
Chemical control – There are several synthetic insecticides that are effective against thrips. It is important to rotate chemicals among different mode of action groups to reduce the risk of insecticide resistance. The following are some synthetic active ingredients and their mode of actions groups in parenthesis that can be used for thrips control: methomyl (1A), bifenthrin (3A), lambda-cyhalothrin (3A), zeta-cypermethrin (3A), clothianidin (4A), spinetoram (5), and cyantraniliprole (28).
Depending on the level of control needed, combinations of products from different categories can improve control efficacy. For example, a combination of entomopathogenic fungi and nematodes can be applied to the soil for controlling prepupae and pupae. While the soil-dwelling predatory mite S. scimitus and the rove beetle, D. coriaria, can be used against pupal stages, other natural enemies can be used against nymphs and adults. A combination of entomopathogenic fungi and azadirachtin can be applied both to the soil or foliage for controlling different life stages. Similarly, various biological and synthetic insecticides can be applied in combination or rotation to obtain desired control.
The categories presented above are based on the source or nature of the active ingredients and do not indicate their organic or conventional label status. Please check the product labels for their appropriateness for managing thrips in lettuce, for use in organic farms, and guidelines for storage, handling, and field use. Entomopathogenic nematodes, fungi, and other biologicals are compatible with several synthetic agricultural inputs, but verify the label guidelines for specific instructions.
Additional resources:
Dara, S. K. 2019. The new integrated pest management paradigm for the modern age. JIPM 10: 1-9. https://doi.org/10.1093/jipm/pmz010
Dara, S. K. 2021. Biopesticides: categories and use strategies for IPM and IRM. UC ANR eJournal of Entomology and Biologicals. https://ucanr.edu/blogs/blogcore/postdetail.cfm?postnum=46134
Natwick, E. T., S. V. Joseph, and S. K. Dara. 2017. UC IPM pest management guidelines: lettuce. UC ANR Publication 3450. https://www2.ipm.ucanr.edu/agriculture/lettuce/Western-flower-thrips/
Riley, D. G., S. V. Joseph, R. Srinivasan, and S. Diffie. 2011. Thrips vectors of tospoviruses. JIPM 2: I1-I10. https://doi.org/10.1603/IPM10020
- Author: Surendra K. Dara
- Author: Roland C. Bocco
The spotted lanternfly (SLF) [Lycorma delicatula (Hemiptera: Fulgoridae)] is an invasive planthopper, which causes a significant damage to apples, grapes, stone fruit, trees used for timber, and other hosts (Dara et al. 2015). Native to China, SLF was first reported in 2014 in Pennsylvania and has been rapidly spreading in the eastern United States and moving westward. California has 22 cultivated and about 70 wild hosts of SLF and include several high value crops such as apples, cherries, grapes, and plums. The tree-of-heaven, an invasive species, is a favorite host of SLF and is widely distributed in California. SLF is also a nuisance pest with 100s or 1000s of individuals infesting landscape trees and hosts in residential areas. This pest deposits eggs on inanimate objects such as vehicles, furniture, stones, and packages and thus spread to other areas through the movement of these objects. Awareness of the pest and its damage potential, ability of Californians to recognize and report the pest if found, and the knowledge of control practices will help prevent accidental transportation of eggs or other life stages from the infested areas to California and prepare the citizens to take appropriate actions. Outreach efforts have been made in California since 2014 through extension articles, presentations at extension meetings, videos, social media posts, and personal communication (Dara, 2014).
Wakie et al. (2020) modeled the establishment risk of SLF in the United States and around the world and indicated that many coastal regions and the Central Valley of California are among the high-risk areas. Considering the risk to several high-value commodities and the presence of several wild hosts that are distributed all over California, mapping of the risk-prone areas based on the cultivated hosts, their acreage and value in different counties, and the distribution of wild hosts was done to help both growers and other Californians to prepare for potential invasion of SLF.
Methodology
The list of SLF hosts is continuously evolving with host specificity studies in various places. Based on two published resources (Dara et al. 2015; Barringer and Ciafré 2020), 22 cultivated and 70 wild hosts appear to be present in California. Plant species that support some of the feeding life stages or all life stages were included in preparing these lists. The cultivated hosts include apples, apricots, basil, blueberries, butternut, cherries, cotton, grapes, hibiscus, hops, mock orange, nectarine, peaches, pears, persimmon, plums, pomegranates, roses, soybean, sponge gourd, tea, and walnuts; and the wild hosts include Acacia sp., American hazelnut, Amur corktree, American linden, American sycamore, arborvitae, Argentine cedar, Asian white birch, bee balm, big-toothed aspen, black gum, black hawk, black locust, black walnut, Bladder senna, boxelder, chestnut oak, chinaberry tree, Chinese boxwood, Chinese juniper, Chinese parasol tree, Chinese wingnut, devilwoods, dogwood, Eastern white pine, edible fig, false spiraea, fireweed, five-stamen tamarisk, flowering dogwood, Forsythia, Glossy privet, greater burdock, grey alder, hemp, hollyhocks, honeysuckle, hornbeam, Japanese angelica, Japanese boxwood, Japanese maple, Japanese snowball, Japanese zelkova, jujubes, Kobus magnolia, Northern spicebush, Norway maple, lacquer tree, perennial salvia, Persian silk tree, plane tree, Poinsettia, poplars, princess tree, red maple, sapphire dragon tree, sassafras , sawtooth, serviceberry, silver maple, slippery elm, snowbell, staghorn sumac, sugar maple, tree-of-heaven, tulip tree, Virginia creeper, white ash, wild grape, and willows.
The summary of county crop reports from the California Department of Food and Agriculture (CDFA 2018) was used to determine the value and acreages of the cultivated hosts. To determine the distribution of wild hosts various online resources were used. SLF risk levels were determined as very low, low, moderate, high, and very high for the number of hosts, acreage and value of each cultivated host, and other such parameters within each county. The highest risk value within each parameter was used to determine ‘very high' category and 4/5, 3/5, 2/5, and 1/5 were used for high, moderate, low, and very low categories, respectively. In other words, 0-20% risk was considered very low, 21-40% as low, 41-60% as moderate, 61-80% as high, and 81-100% as very high for each measured parameter. Data were entered into a spreadsheet and maps were generated using QGIS open-source cross-platform geographic information system application.
Risk-prone areas in California
The following maps show areas in California that are prone to SLF risk based on the distribution of cultivated and wild hosts, and the acreage and value of important cultivated crops.
Based on these maps, the entire state of California is at some level of risk. In addition to the commercially produced crops, several backyard or landscape plant species such as roses, grapes, peaches, plums, and others are present throughout the state and can harbor SLF. Such host plants in residential and urban landscapes can serve as SLF sources for commercial crops. The tree-of-heaven is present throughout California and several such uncultivated hosts can serve as sources of undetected infestations. While researchers are working on appropriate biocontrol solutions such as releasing natural enemies, other control options such as synthetic and microbial pesticide applications, sticky traps, removal of egg masses and wild hosts, and other strategies can help manage SLF. In the meantime, Californians will benefit by knowing about this pest and its potential risk to the state. The ability to identify, destroy or capture, and report the pest to county and state departments or University of California Cooperative Extension offices will help prevent or delay SLF invasion and spread in California.
Conclusion
California is at the risk of SLF invasion and spread. Depending on the number of cultivated crops, their acreage, value, and the distribution of wild hosts, the risk level varies in various counties throughout the state. Outreach efforts are helping to alert Californians about SLF and its damage to cultivated crops and nuisance in urban and residential areas.
Additional resources
Refer to https://ucanr.edu/spottedlanternfly for additional information about the pest. If you happen to see this pest in California, please contact your local Agricultural Commissioner, California Department of Food and Agriculture, or UC Cooperative Extension office to report.
Acknowledgments
Thanks to the California Department of Food and Agriculture for funding this study.
References
Barringer, L. and Ciafré, C. M. 2020. Worldwide feeding host plants of spotted lanternfly, with significant additions from North America. Environ. Entomol. 49: 999-1011.
CDFA (California Department of Food and Agriculture). 2018. California County Agricultural Commissioners' Report Crop Year 2016-2017 (https://www.cdfa.ca.gov/statistics/pdfs/2017cropyearcactb00.pdf).
Dara, S. K. 2014. Spotted lanternfly (Lycorma delicatula) is a new invasive pest in the United States. UCANR eJournal Pest News (https://ucanr.edu/blogs/blogcore/postdetail.cfm?postnum=15861).
Dara, S. K. 2018. An update on the invasive spotted lanternfly, Lycorma delicatula: current distribution, pest detection efforts, and management strategies. UCANR eJournal Pest News (https://ucanr.edu/blogs/blogcore/postdetail.cfm?postnum=26349).
Dara, S. K., Barringer, L. and Arthurs, S. P. 2015. Lycorma delicatula (Hemiptera: Fulgoridae): a new invasive pest in the United States. J. Integr. Pest Manag. 6: 20.
Wakie, T. T., Nevin, L. G., Yee, W. L. and Lu, Z. 2020. The establishment risk of Lycorma delicatula (Hemiptera: Fulgoridae) in the United States and globally. J. Econ. Entomol. 113: 306-314.
- Author: Surendra K. Dara
- Author: Carson DiCicco, Vina Quest
The western grapeleaf skeletonizer (WGLS), Harrisina metallica, is a pest of vineyards in some parts of California. Larval feeding skeletonizes grape leaves and uncontrolled populations can lead to a complete loss of foliage, fruit damage, and yield reduction. WGLS populations are usually suppressed with standard pest management practices used against it or other pests. However, considering regular WGLS infestations in the past few years especially in organic vineyards in warmer parts of the state warrant development of a good monitoring and integrated pest management strategy to improve the pest control efficacy and to minimize the risk of resistance development from potential overuse of limited organic pesticides. An earlier bioassay with biologicals showed azadirachtin, spinosad, Bacillus thuringiensis subsp. aizawai, and entomopathogenic fungi Beauveria bassiana and Metarhizium sp. as potential control options (Dara et al., 2019). The potential of Harrisina brillians granulovirus, a naturally occurring virus that previously suppressed WGLS populations a few decades ago is also explored as a natural solution (Federici and Stern, 1990).
WGLS has 2-3 generations per year with late spring-early summer and mid-late summer infestations in the coastal regions (Fig. 1). Based on the detection of shiny black moths and growing degree-day calculations, pesticide applications can be timed to target hatching larvae. Growing degree-day calculations were made using a model provided by Pest Prophet and temperature data from GreenCast. Good monitoring tools such as traps equipped with lures can be useful to improve the monitoring accuracy especially when the adult activity spreads over multiple weeks for each generation. A study was conducted to assist with the development of new lures for WGLS.
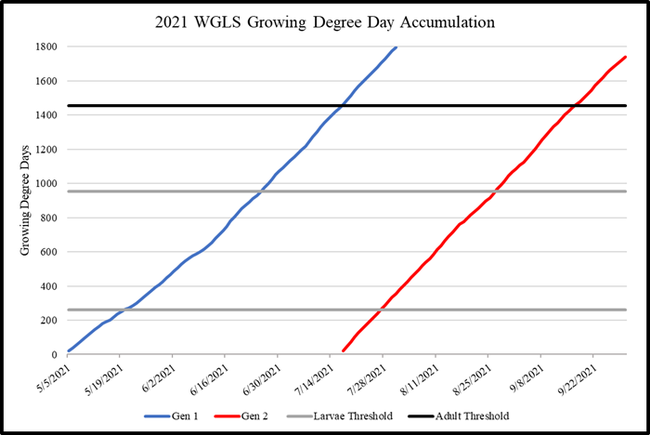
Fig. 1. Growing degree-days indicating WGLS sping and summer generations and thresholds for larvae and adults
Methodology
An organic Cabernet Sauvignon vineyard (San Juan North) in Shandon was used for the study conducted between May and July 2021. Treatments included a blank lure, Pherocon WGLS, TRE 2500, and TRE 2501. The last two are developmental formulations. The pheromone components of the lures are different combinations of 2S-butyl Z7-tetradecenoate, 2-butyl decanoate, 2-butyl dodecanoate, and isopropyl Z7-tetradecenoate to attract male moths and the latter two are new combinations of active ingredients. Each treatment was replicated six times in a randomized complete block design. Within each treatment, a lure was placed in Pherocon VI Delta trap with an adhesive, replaceable liner and tied in the top part of the canopy. A 30 m distance was maintained between the traps with and between replications. Traps were first set up with new lures and liners on 8 May 2021. Adhesive liners were observed every week between 15 May and 3 July 2021 on eight observation dates to count the number of moths. Lures were replaced on 5 June 2021 and adhesive liners were replaced every week or every other week as needed. Data were analyzed using Statistix software and Tukey's HSD test was used to separate significant means.
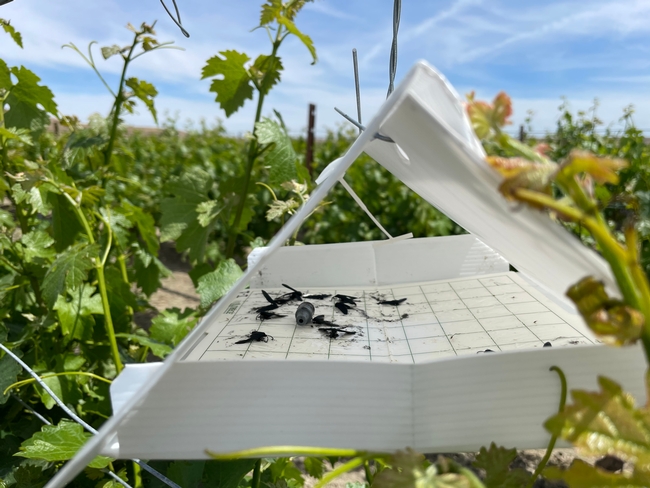
Pheromone infused lure surrounded by the western grapeleaf sekeltonizer male moths (Above photo by Surendra Dara and below photo by Carson DiCicco)
Results
Moth counts significantly (P < 0.0005) varied among the lures on all observation dates (Table 1 and Fig. 2). In general, TRE 2501 lure attracted significantly higher number of moths for most of the observation period. Due to a logistics issue, adhesive liners were not replaced after the moth counts were made on 12 June and those numbers were detected from the next count to derive 19 June moth counts. A lack of space on the liner was probably the reason for having lower moth numbers on 19 June 2021 in TRE 2501. Pherocon WGLS, which is commercially available in the market, was generally less attractive than the developmental formulations. Pheromone combination in the TRE 2501 can be considered for the new formulation for improved monitoring efficacy. Compared to visual monitoring of moth activity, using lures appeared to be an effective strategy for monitoring WGLS, which helped the grower to make effective treatment decisions. On average, 287 moths were captured per each TRE 2501 lure during the 8-week observation period. Considering that each moth can deposit 300 eggs in its lifetime, trapping adults during monitoring can also contribute to reduction in their offspring. In addition to serving as a monitoring tool, lures can also be a control option, if economical.
Acknowledgments: Thanks to Trece for providing lures and traps for the study.
References
Dara, S. K., S. S. Dara, and S. Jaronski. 2019. Biorational control options for the western grapeleaf skeletonizer, a re-emerging pest in California. eJournal of Entomology and Biologicals. https://ucanr.edu/blogs/blogcore/postdetail.cfm?postnum=29081
Federici, B. A. and V. M. Stern. 1990. Replication and occlusion of a granulosis virus in larval and adult midgut epithelium of the western grapeleaf skeletonizer, Harrisina brillians. J. Invertebr. Pathol. 56: 401-414.
- Author: Surendra K. Dara
Biopesticides contain active ingredients of natural or biological origin that include plant extracts, microorganisms, microbial metabolites, organic molecules, minerals, or other such natural materials that have pesticidal properties. Pests such as herbivorous arthropods, pathogens, parasitic nematodes, mollusks, rodents, and weeds cause significant crop damage when they are not managed. Pest suppression is a critical part of crop production to maintain plant health, prevent yield losses, and optimize returns. As agriculture advanced from subsistence farming to a global enterprise, crop protection also evolved over millennia. When farming was less organized, nature maintained a balance and provided solutions initially. Then natural solutions were actively implemented until industrialization led to the use of synthetic inputs in the 20th century. While synthetic fertilizers and pesticides contributed to a tremendous improvement in the yield potential, the indiscriminate use of some of them and the resulting damage to the environment and human health steered food production in the recent past towards organic farming with the use of nature-based solutions.
Although biopesticides have been around for a few decades, the growth of organic farming gave an impetus to the biopesticide industry during the past few years resulting in the development of new active ingredients and improved formulations. Now, biopesticides are considered an important part of integrated pest management (IPM) strategies in both organic and conventional systems. With a considerable industry investment in research and development, the quality and efficacy of biopesticides have also significantly improved. This has also contributed to optimizing the cost of some formulations. However, there is still a need to fill the knowledge gaps in biopesticides and their use. Depending on the active ingredient, the mode of action for biopesticides, their target pests, their storage and handling, and the use strategies are quite diverse, and a thorough understanding of these aspects is critical for their successful use. As emphasized in the new IPM model (Dara, 2019), while biopesticide use is an integral part of crop protection, understanding the pest biology, using biopesticides appropriate for the target life stage of the pest, applying them at the right time and rate using the right technology, avoiding incompatibility issues, building and sharing effective use strategies, and continuously investing in research and outreach are essential elements of biopesticide use. Biopesticides also play an important role in insecticide resistance management (IRM) to address resistance issues associated with synthetic pesticides. This article provides an overview of various biopesticide categories and general strategies for their successful use for IPM and IRM.
Biopesticides can be used for managing arthropod pests, bacterial or fungal pathogens, plant-parasitic nematodes, weeds, and snails and slugs. Some formulations or active ingredients have multiple roles and can be effective against more than one category of pests. While some active ingredients are very specific to a particular pest or related species, others have a broad-spectrum activity. Based on the source, biopesticides can be placed in four broad categories: i) botanicals, ii) microbials, iii) toxins, and iv) minerals and other natural materials.
Botanical extracts: Plants are a rich source of numerous phytochemicals or secondary metabolites that have a wide range of properties including pesticidal activity. Acids, alkaloids, flavonoids, glycosides, saponins, and terpenoids in plant extracts or oils obtained from seeds and other plant parts are some of the compounds present in various biopesticides (Pino et al., 2013). Azadirachtin, BLAD (polypeptide from sweet lupine seeds), citric acid, essential oils, pyrethrins, soybean oil, and extract of the giant knotweed are used for their acaricidal, insecticidal, fungicidal, nematicidal, or herbicidal properties.
Microbials: Some of the microbial pesticides have live microorganisms (such as entomopathogens, Bacillus spp., Streptomyces spp., and Trichoderma spp.) while others (such as Burkholderia rinojensis and Chromobacterium subtsugae)have heat-killed microorganisms and fermentation solids as the active ingredients. Entomopathogenic microorganisms [Bacillus thuringiensis (bacterium), Beauveria bassiana and Cordyceps fumosorosea (fungi), Heterorhabditis spp. and Steinernema spp. (nematodes), and granuloviruses and nucleopolyhedroviruses] primarily kill their hosts through infection; microbe-based fungicides antagonize plant pathogens through competitive displacement and production of toxic metabolites; nematophagous fungi parasitize plant-parasitic nematodes; and plant pathogenic bacteria, fungi, and viruses infect and suppress weeds. Bacteriophages, which are viruses that parasitize bacteria, are used against the plant pathogenic species of Clavibacter, Erwinia, Pseudomonas, Xanthomonas, Xylella, and other genera.
Toxins and other organic molecules: There are multiple examples of toxic organic molecules derived from various organisms. Avermectins from the bacterium Streptomyces avermitilis and spinosad from the bacterium Saccharopolyspora spinosa, strobilurin from the mushroom Strobuluris tenacellus, and cerevisane from the yeast Saccharomyces cerevisae are some of the microbial toxins that are effective against insects, plant-parasitic nematodes, or snails and slugs. A venom peptide from the Blue Mountains funnel-web spider, Hadronyche versuta, from Australia is a recently developed insecticide active ingredient with its unique mode of action class. Chitosan, a polysaccharide from the exoskeleton of shellfish, is a fungicide.
Minerals and other natural materials: Diatomaceous earth, mineral oil, and minerals such as sulfur are used for controlling multiple categories of pests. Potassium salts of fatty acids of plant or animal origin, known as insecticidal soap, have insecticidal and fungicidal properties. Organic acids such as acetic acid and citric acid are derived from plants and have fungicidal and herbicidal properties. Since these are different from other botanical extracts, they are placed in this category.
Except for the microbial pesticides that have live microorganisms, most biopesticides have chemical molecules of microbial, fungal, botanical, or mineral origin and work through various modes of action similar to synthetic pesticides. Several synthetic pesticides are developed from natural molecules. Abamectin, pyrethroids, neonicotinoids, spinetoram, and storbulurins are synthetic analogs based on avermectins, pyrethrins, nicotine, spinosad, and strobulurin, respectively, and were developed for improved stability, safety, or ease of commercial-scale production.
Integrated pest management and resistance management: Biopesticides are very diverse in their origin and mode of action and have been successfully used in several cropping systems for managing a variety of pests. They have complex interactions with plants, soil microbiota, pests, and environmental conditions. It is critical to have a good understanding of the source of biopesticides and how they act on their target pests. Certain biopesticides may have special storage and handling requirements or tank-mixing restrictions. It is essential to refer to the manufacturer's guidelines or label instructions to avoid incompatible tank-mix combinations, understand proper application sequences, and to store, transport, and apply under unfavorable conditions. While it is very important to use biopesticides as a part of the IPM program and tools for IRM, caution is warranted to avoid repeated use of the same or a similar type of biopesticide. Pests can develop resistance to biopesticides just as they do to synthetic pesticides (Dara, 2020).
Strategies for using biopesticides: From the seed or transplant treatment to soil or foliar application, biopesticides can be used throughout crop production. Certain combinations can have an additive or a synergistic effect on pest suppression. At the same time, certain inputs or practices can negatively impact biopesticide efficacy. For example, alkaline tank-mix components breakdown the protein coat of entomopathogenic viruses and Bacillus thuringiensis. Botanical oils can be incompatible with cold water. Some fungicides such as captan and thiram are incompatible with entomopathogenic fungi like Beauveria bassiana while several others are compatible (Dara et al., 2014).
Investing in biopesticides: Environmental safety and resistance development are two major concerns for excessive use of synthetic pesticides and incorporating biopesticides into IPM will help address both issues. Substituting biopesticides for synthetic pesticides will reduce the total amount of the latter during a production season and their potential negative impact on the environment and human health. Several biopesticides are not harmful to pollinators and in some production systems, pollinators are used to deliver biopesticides to the crops they pollinate. Adding biopesticides to the standard crop protection program will also increase pest control efficacy. Additionally, by not continuously using synthetic pesticides, the risk of resistance will be reduced and thus their efficacy will continue to be maintained. Although some biopesticides can be more expensive than synthetic pesticides, investing in them will be a good strategy for both the short-term benefit of effective pest suppression and the long-term benefit of a healthy and resilient ecosystem. Since pests do not have boundaries, area-wide implementation of good agricultural practices with a balanced use of synthetic and natural inputs is necessary for maintaining the productivity of the cropping systems.
Productive collaborations among the pesticide industry, researchers, extension educators, and the grower community are critical for successfully using biopesticides for sustainable food production. While research helps to develop effective formulations and their use strategies, outreach helps with the implementation of those strategies.
References
Dara, S.S.R., S. S. Dara, A. Sahoo, H. Bellam, and S. K. Dara. 2014. Can entomopathogenic fungus Beauveria bassiana be used for pest management when fungicides are used for disease management? UCANR eJournal of Entomology and Biologicals. https://ucanr.edu/blogs/blogcore/postdetail.cfm?postnum=15671
Dara, S. K. 2019. The new integrated pest management paradigm for the modern age. J. Integr. Pest Manag. 10 (1): 12. https://doi.org/10.1093/jipm/pmz010
Dara, S. K. 2020. Arthropod resistance to biopesticides. Organic Farmer 3 (4): 16-19. https://organicfarmermag.com/2020/08/arthropod-resistance-to-biopesticides/
Pino, O. Y. Sánchez, and M. M. Rojas. 2013. Plant secondary metabolites as an alternative in pest management. I: Background, research approaches and trends. Rev. ProtecciónVeg. 28 (2): 81-94.