- Author: Ben Faber
Like most of us, trees don't want to be eaten alive.
To prevent this gruesome fate, they developed extremely tough cell walls around 400 million years ago. For millions of years, nothing could break down lignin, the strongest substance in those cell walls. When a tree died, it just sank into the swamp where it grew. When the fossil record started showing trees breaking down around 300 million years ago, most scientists assumed it was because the ubiquitous swamps of the time were drying up.
But biologist David Hibbett at Clark University suspected that wasn't the whole story. An alternative theory from researcher Jennifer Robinson intrigued him. She theorized that instead of ecosystem change alone, something else played a major role - something evolving the ability to break down lignin. Through evolutionary biology research supported by the Department of Energy's (DOE) Office of Science, Hibbett and his team confirmed her theory. They found that, just as she predicted, a group of fungi known as "white rot fungi" evolved the ability to break down lignin approximately the same time that coal formation drastically decreased. His research illustrated just how essential white rot fungi were to Earth's evolution.
Fungi are still indispensable. The short-order cooks of the natural world, they have an unheralded job making nutrients accessible to the rest of us. Just like cooking spinach makes it easier to digest, some fungi can break down plant cell walls, including lignin. That makes it easier for other organisms to use the carbon that is in those cell walls.
"We all live in the digestive tract of fungi," said Scott Baker, a biologist at DOE's Pacific Northwest National Laboratory. If we weren't surrounded by fungi that decay dead plant material, it would be much harder for plants to obtain the nutrients they need.
To understand fungi's role in the ecosystem and support biofuels research, scientists supported by DOE's Office of Science are studying how fungi have evolved to decompose wood and other plants.
The Special Skills of Fungi
Fungi face a tough task. Trees' cell walls contain lignin, which holds up trees and helps them resist rotting. Without lignin, California redwoods and Amazonian kapoks wouldn't be able to soar hundreds of feet into the air. Trees' cell walls also include cellulose, a similar compound that is more easily digested but still difficult to break down into simple sugars.
By co-evolving with trees, fungi managed to get around those defenses. Fungi are the only major organism that can break down or significantly modify lignin. They're also much better at breaking down cellulose than most other organisms.
In fact, fungi are even better at it than people and the machines we've developed. The bioenergy industry can't yet efficiently and affordably break down lignin, which is needed to transform non-food plants such as poplar trees into biofuels. Most current industrial processes burn the lignin or treat it with expensive and inefficient chemicals. Learning how fungi break down lignin and cellulose could make these processes more affordable and sustainable.
Tracing the Fungal Family Tree
While fungi live almost everywhere on Earth, advances in genetic and protein analysis now allow us to see how these short-order cooks work in their kitchen. Scientists can sample a fungus in the wild and analyze its genetic makeup in the laboratory.
By comparing genes in different types of fungi and how those fungi are evolutionarily related to each other, scientists can trace which genes fungi have gained or lost over time. They can also examine which genes an individual fungus has turned "on" or "off" at any one time.
By identifying a fungus's genes and the proteins it produces, scientists can match up which genes code for which proteins. A number of projects seeking to do this tap the resources of the Joint Genome Institute (JGI) and the Environmental Molecular Sciences Laboratory (EMSL), both Office of Science user facilities.
Understanding the Rot
Just as different chefs use different techniques, fungi have a variety of ways to break down lignin, cellulose, and other parts of wood's cell walls.
White Rot
Although fungi appeared millions of years earlier, the group of fungi known as white rot was the first type to break down lignin. That group is still a major player, leaving wood flaky and bleached-looking in the forest.
"White rot is amazing," said Hibbett.
To break down lignin, white rot fungi use strong enzymes, proteins that speed up chemical reactions. These enzymes split many of lignin's chemical bonds, turning it into simple sugars and releasing carbon dioxide into the air. White rot is still better at rending lignin than any other type of fungus.
Brown Rot
Compared to white rot's powerful effects, the scientific community long thought the group known as brown rot fungi was weak. That's because brown rot fungi can't fully break down lignin.
Recalling his college classes in the 1980s, Barry Goodell, a professor at the University of Massachusetts Amherst said, "Teachers at the time considered them these poor little things that were primitive."
Never underestimate a fungus. Even though brown rot fungi make up only 6 percent of the species that break down wood, they decompose 80 percent of the world's pine and other conifers. As scientists working with JGI in 2009 discovered, brown rot wasn't primitive compared to white rot. In fact, brown rot actually evolved from early white rot fungi. As the brown rot species evolved, they actually lost genes that code for lignin-destroying enzymes.
Like good cooks adjusting to a new kitchen, evolution led brown rot fungi to find a better way. Instead of unleashing the brute force of energy-intensive enzymes alone, they supplemented that enzyme action with the more efficient "chelator-mediated Fenton reaction" (CMF) process. This process breaks down wood cell walls by producing hydrogen peroxide and other chemicals. These chemicals react with iron naturally in the environment to break down the wood. Instead of fully breaking down the lignin, this process modifies it just enough for the fungus to reach the other chemicals in the cell wall.
There was just one problem with this discovery. In theory, the CMF chemical reaction is so strong it should break down both the fungus and the enzymes it relies on. "It would end up obliterating itself," said Jonathan Schilling, an associate professor at the University of Minnesota.
Scientists' main theory was that the fungus created a physical barrier between the reaction and the enzymes. To test that idea, Schilling and his team grew a brown rot fungus on very thin pieces of wood. As they watched the fungus work its way through the wood, they saw that the fungus was breaking up the process not in space, but in time. First, it expressed genes to produce the corrosive reaction. Two days later, it expressed genes to create enzymes. Considering fungi can take years or even decades to break down a log, 48 hours is a blip in time.
Scientists are still trying to figure out how much of a role the CMF process plays. Schilling and like-minded researchers think enzymes are still a major part of the process, while Goodell's research suggests that CMF reactions do most of the work. Goodell's team reported that CMF reactions could liquefy as much as 75 percent of a piece of pine wood.
Either way, the CMF process offers a great deal of potential for biorefineries. Using brown rot fungi's pretreatment could allow industry to use fewer expensive, energy-intensive enzymes.
A Close Collaboration
Not all fungi stand alone. Many types live in symbiosis with animals, as the fungus and animal rely on each other for essential services.
Partnerships with Rumens
Cows and other animals that eat grass depend on gut fungi and other microorganisms to help break down lignin, cellulose, and other materials in wood's cell walls. While fungi only make up 8 percent of the gut microbes, they break down 50 percent of the biomass.
To figure out which enzymes the gut fungi produce, Michelle O'Malley and her team at the University of California, Santa Barbara grew several species of gut fungi on lignocellulose . They then fed them simple sugars. As the fungi "ate" the simple sugars, they stopped the hard work of breaking down the cell walls, like opting for take-out rather than cooking at home.
Depending on the food source, fungi "turned off" certain genes and shifted which enzymes they were producing. Scientists found that these fungi produced hundreds more enzymes than fungi used in industry can. They also discovered that the enzymes worked together to be even more effective than industrial processes currently are.
"That was a huge diversity in enzymes that we had never seen," said O'Malley.
O'Malley's recent research shows that industry may be able to produce biofuels even more effectively by connecting groups of enzymes like those produced by gut fungi .
Termites as Fungus Farmers
Some fungi work outside the guts of animals, like those that partner with termites. Tropical termites are far more effective at breaking down wood than animals that eat grass or leaves, both of which are far easier to digest. Young termites first mix fungal spores with the wood in their own stomachs, then poop it out in a protected chamber. After 45 days of fungal decomposition, older termites eat this mix. By the end, the wood is almost completely digested.
"The cultivation of fungus for food [by termites] is one of the most remarkable forms of symbiosis on the planet," said Cameron Currie, a professor at the University of Wisconsin, Madison and researcher with the DOE's Great Lakes Bioenergy Research Center.
Scientists assumed that the majority of the decomposition occurred outside of the gut, discounting the work of the younger termites. But Hongjie Li, a biologist at the University of Wisconsin, Madison, wondered if younger insects deserved more credit. He found that young workers' guts break down much of the lignin. In addition, the fungi involved don't use any of the typical enzymes white or brown rot fungi produce. Because the fungi and gut microbiota associated with termites have evolved more recently, this discovery may open the door to new innovations.
From the Lab to the Manufacturing Floor
From the forest floor to termite mounds, fungal decomposition could provide new tools for biofuels production. One route is for industry to directly produce the fungal and associated microbiota's enzymes and other chemicals. When they analyzed termite-fungi systems, scientists found hundreds of unique enzymes.
"We're trying to dig into the genes to discover some super enzyme to move into the industry level," said Li.
A more promising route may be for companies to transfer the genes that code for these enzymes into organisms they can already cultivate, like yeast or E. coli. An even more radical but potentially fruitful route is for industry to mimic natural fungal communities.
For millions of years, fungi have toiled as short-order cooks to break down wood and other plants. With a new understanding of their abilities, scientists are helping us comprehend how essential they are to Earth's past and future.
Photo:
The evolution of white rot fungi most likely played a large role in trees beginning to decay about 300 million years ago. The fungus Schizophyllum commune is one modern example of a white rot fungus. Credit: Photo courtesy of Nathan Wilson

- Author: Ben Faber
PULLMAN, Wash. Soil pathogen testing - critical to farming, but painstakingly slow and expensive - will soon be done accurately, quickly, inexpensively and onsite, thanks to research that Washington State University scientists plant pathologists are sharing.
As the name implies, these tests detect disease-causing pathogens in the soil that can severely devastate crops.
Until now, the tests have required large, expensive equipment or lab tests that take weeks.
The soil pathogen analysis process is based on polymerase chain reaction (PCR) tests that are very specific and sensitive and only possible in a laboratory.
The new methods, designed by WSU plant pathologists, are not only portable and fast, but utilize testing materials easily available to the public. A paper by the researchers lists all the equipment and materials required to construct the device, plus instructions on how to put it all together and conduct soil tests.
Responding to growers needs
"We've heard from many growers that the time it takes to obtain results from soil samples sent to a lab is too long," said Kiwamu Tanaka, assistant professor in WSU's Department of Plant Pathology. "The results come back too late to be helpful. But if they can get results on site, they could make informed decisions about treatments or management changes before they even plant their crop."
Some diseases from soil pathogens may not be visible until weeks after the crop has sprouted, Tanaka said. That could be too late to treat the disease or could force farmers to use more treatments.
Magnetic breakthrough
WSU graduate student Joseph DeShields, a first author on the paper, said it took about six months of work to get their device to work in the field. It relies on magnets to capture pathogens' DNA from the soil.
"It turns out, it's really hard to separate and purify genetic material from soil because soil contains so much material for PCR tests," said DeShields "So we were thrilled when we made that breakthrough."
Rachel Bomberger is a WSU plant diagnostician who helped with the concepts of the machine testing. She said she's impressed by what Tanaka and the team accomplished.
"We removed a huge stumbling block when it comes to soil testing," said Bomberger, one of the co-authors on the paper. "We found the missing piece that makes the testing systems work in the field without expensive lab equipment or testing materials."
Worldwide application
The system was tested on potato fields around eastern Washington, Tanaka said, but it will work on soil anywhere in the world.
"It's a really versatile method," he said. "You could use it for nationwide pathogen mapping or look at the distribution of pathogens around the country. We started small, but this could have huge implications for testing soil health and disease."
Tanaka said it was important for this discovery to be available in an open-access video journal.
"We're always concerned about helping every grower and the industry as a whole," Tanaka said. "We want everybody to look at this and use it, if they think they'll benefit from it."
###
The results were published in the Journal of Visualized Experiments, an open-access journal that includes a video showing how to assemble and used the system and a full list of materials needed to use their method.
This research is supported by the Northwest Potato Research Consortium and the Washington State Department of Agriculture - Specialty Crop Block Grant Program.
See the video here:
And the article here:
https://www.jove.com/pdf/56891/jove-protocol-56891-on-site-molecular-detection-soil-borne-phytopathogens-using-portable
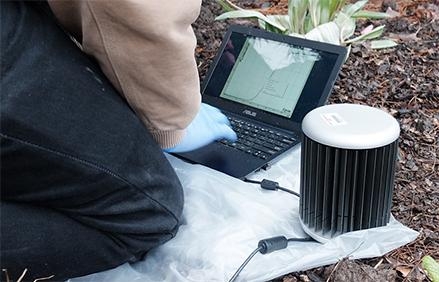
- Author: Ben Faber
The following is the abstract of a recent article highlighting the occurrence of the invasive shot hole borer that is found in California and described in our blog site:
Invasive species are a problem world-wide and this is an example of how invasives can arrive in multiple countries at the same time and/or how possibly they might move from somewhere like California to another far away country like South Africa. People are the usual agents for carrying these pests around the world.
The polyphagous shot hole borer (PSHB) and its fungal symbiontFusarium euwallaceae: a new invasion in South Africa
The polyphagous shot hole borer (PSHB), an ambrosia beetle (Coleoptera: Curculeonidae: Scolytinae) native to Asia, together with its fungal symbiont Fusarium euwallaceae, has emerged as an important invasive pest killing avocado and other trees in Israel and the United States. The PSHB is one of three cryptic species in the Euwallacea fornicatusspecies complex, the taxonomy of which remains to be resolved. The surge in the global spread of invasive forest pests such as the PSHB has led to the development of programs utilizing sentinel tree plantings to record new host-pest interactions. During routine surveys of tree health in botanical gardens of South Africa undertaken as part of a sentinel project, an ambrosia beetle/fungal associate was detected damaging Platanus x acerifolia(London Plane) in the KwaZulu-Natal National Botanical Gardens, Pietermaritzburg.
Identification of the beetle by sequencing part of the mitochondrial cytochrome oxidase c subunit 1 (COI) gene confirmed its identity as PSHB, and specifically one of the invasive haplotypes of the beetle. The associated fungus F. euwallaceaewas identified based on phylogenetic analysis of elongation factor (EF 1-α) sequences. Koch's postulates have confirmed the pathogenicity of fungal isolates toP. x acerifolia. This is the first report of PSHB and its fungal symbiont causing Fusarium dieback in South Africa. This report also represents the first verified case of a damaging invasive forest pest detected in a sentinel planting project, highlighting the importance of such studies. Given the potential impact these species present to urban trees, native biodiversity and agriculture, both the PSHB and its fungal symbiont should be included in invasive species regulations in South Africa.
The full paper is at:
https://doi.org/10.1007/s13313-018-0545-0
Photo: Infected sycamore which is related to London plane tree.
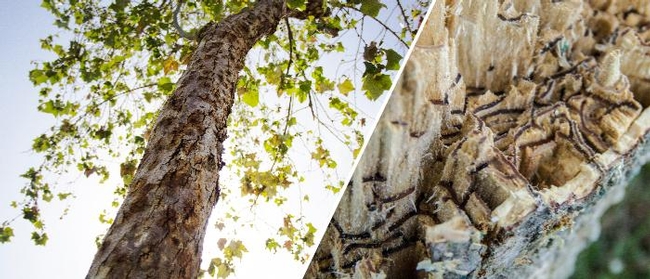
- Author: Ben Faber
No rain for several years may have let citrus growers think that brown rot control is not important. But it still is and it's just coming out after a few inches of rain earlier this January. This is not the brown rot of stone fruits caused by Monilina fungus but a pathogen, completely different – Phytophthora spp.
Symptoms appear primarily on mature or nearly mature fruit. Initially, the firm, leathery lesions sometimes have a water-soaked appearance. Lesions are tan to olive brown, have a pungent odor, and may turn soft from secondary infections. Infected fruit eventually drop. Occasionally, twigs, leaves, and blossoms are infected, turning brown and dying.
Brown rot is caused by multiple species of Phytophthora when conditions are cool and wet. Brown rot develops mainly on fruit growing near the ground when Phytophthora spores from the soil are splashed onto the tree skirts during rainstorms; infections develop under continued wet conditions. Fruit in the early stage of the disease may go unnoticed at harvest and infect other fruit during storage.
Brown rot management primarily relies on prevention. Pruning tree skirts 24 or more inches above the ground can significantly reduce brown rot.
One spray of copper fungicide between October and December before or just after the first rain may provide protection throughout the wet season. When rainfall is excessive, you may have to repeat the spray in January or February. Spray the skirts to about 4 feet above ground. Spraying the ground underneath the trees also reduces brown rot infections. In addition to copper, other products effective against brown rot include the phosphonate and phenylamide fungicides. Phosphonates are applied as foliar and fruit or soil treatments, whereas phenylamides are applied as soil treatments for brown rot control. For soil applications, microsprinkler irrigation applications may be used.
Recently, oxathiapiprolin (Orondis®) was registered for use on citrus in California. Orondis® is an outstanding alternative for Phytophthora control that may be applied as chemigation or foliar application. However, foliar applications are preferred for preventing brown rot.
Postharvest Packinghouse Treatments to Prevent Fruit Decay
Potassium phosphite fungicides may be applied in aqueous dilutions to fruit alone or in combination with other postharvest fungicide treatments to manage nonvisible infections that occurred before harvest or protect fruit from brown rot infection after harvest during storage, distribution, and marketing. Use high-volume flooder or dip treatments for maximum coverage of fruit. Heated (125–136°F) fungicide solutions optimize performance of the potassium phosphite treatment.
More citrus brown rot information is at:
http://ipm.ucanr.edu/PMG/r107100711.html
Packers should check the Global MRL Database for all country MRLs at https://globalmrl.com/db#pesticides/query.
Photos of brown rot on different citrus varieties - lemon, mandarin, orange
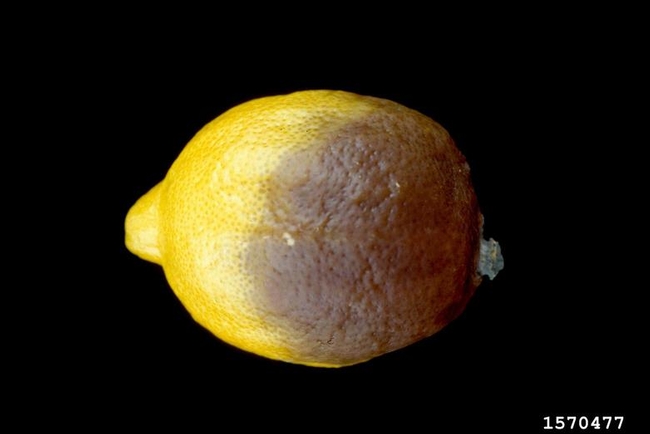
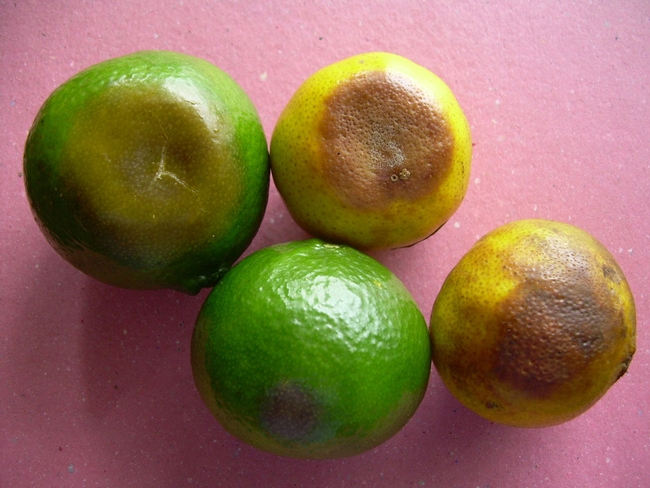
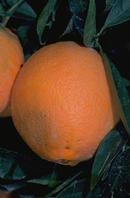
- Author: Steve Tjosvold and Steve Koike
Diseases, disorders and other plant problems are critical concerns for the wholesale nursery. These include biotic problems — caused by living organisms such as pathogens, nematodes, and insects and other arthropods — as well as abiotic problems — caused by factors such as temperature and moisture extremes, mechanical damage, chemicals,
nutrient deficiencies or excesses, salt damage and other environmental factors. Many plant problems, especially biotic problems, if not recognized and controlled early in their development, can result in significant economic damage for the producer. Therefore, timely and accurate diagnoses are required so that appropriate pest and disease
management options and other corrective measures can be implemented.
Definition of Plant Diagnosis and Steps
Diagnosis is the science and art of identifying the agent or cause of the problem under investigation. When one renders a diagnosis, one has collected all available information, clues and observations and then arrives at an informed conclusion as to the causal factor(s). Hence, plant problem diagnosis is an investigative, problem-solving process that involves the following steps:
- Ask and answer the appropriate questions to define the problem and
obtain information that is relevant to the case under investigation.
- Conduct a detailed, thorough examination of the plants and production areas.
- Use appropriate field diagnostic kits and lab tests to obtain clinical information on possible causal agents and factors.
- Compile all the collected information and consult additional resources and references.
- Finally, make an informed diagnosis.
Throughout this process compile all notes, observations, maps, laboratory results, photographs and other information. This compilation will be the information base for the present diagnosis and can also be a useful resource for future diagnostic cases. Keep an open mind as the information is analyzed and do not make unwarranted assumptions.
Distinguishing Abiotic and Biotic Problems
The first step is to determine whether the problem is caused by an infectious agent, and this can be difficult. Plant symptoms caused by biotic factors such as infectious diseases and arthropod pests are oftensimilar to damage caused by other factors. Leaf spots, chlorosis, blights, deformities, defoliation, wilting, stunting and plant death can
be common symptoms of both biotic and abiotic problems; therefore, the presence of these symptoms does not necessarily mean the problem is a disease. Some general guidelines for distinguishing abiotic and biotic
problems follow and are summarized in table 1.
Table 1 DISTINGUISHING ABIOTIC AND BIOTIC PROBLEMS |
||
Characteristics |
Abiotic |
Biotic |
Hosts |
often affects several species or plants of various ages |
often affects one species or cultivar of the same age |
Pattern of plant symptoms |
often related to environmental or physical factors or cultural practices; may be regular or uniform |
often initially observed in random or irregular locations |
Rate of symptom development |
relatively uniform, extent of damage appears similar among plants |
relatively uneven, time of appearance and damage severity varies among affected plants |
Signs |
no evidence of the kinds of pests or pathogens known to cause the current symptoms |
presence of insects, mites, |
Spread |
is not infectious, is not progressive, commonly caused by one incident and does not spread |
infectious, spreads on host over time if environmental conditions are suitable |
Recurrence |
possibly previously associated with current or prior environmental conditions or cultural practices |
possibly caused by pests that |
Adapted from Table 18, ANR Pub 3420 |
Biotic problems. Identifying biotic problems is sometimes facilitated if signs of a pathogen, primarily the growth of a fungus, are present. The most obvious examples of such signs are the mycelium and spores produced by rusts and powdery and downy mildews. However, in other cases nonpathogenic fungican grow on top of damaged plant tissues and appear to be signs of a pathogen, resulting in possible misdiagnoses.
Biotic problems often affect one species or cultivar of the same age and typically are initially observed in random or irregular locations; symptoms appear at varying times, and severity varies among affected plants. Biotic problems are infectious, spreading when environmental conditions are favorable, and may be associated with pests that have affected the crop. This infectious aspect is important, as biotic diseases will many times be progressive and continue to affect
additional tissues and more plants.
Abiotic problems. In contrast to biotic factors, abiotic problems often affect several species or plants of various ages; typically, damage is relatively uniform, doesn't spread and is often not progressive. Abiotic problems are not associated with pests. They are often caused by a single incident and are related to environmental or physical factors or cultural practices. Once the responsible factor has dissipated and is no longer affecting the plant, the plant may grow out of the problem and develop new, normal appearing foliage.
Diagnosing Biotic Problems
Infectious diseases. To confirm if a problem is caused by a pathogenic fungus, bacterium, nematode, or virus, it is often necessary to have symptomatic tissues analyzed by a trained horticulturalist or plant pathologist. Such experts will attempt to microscopically observe the agent and recover it, if culturable, through isolation procedures. Lab analysis is particularly important to determine if multiple pathogens are infecting the plant. A downside is that obtaining a diagnosis from lab analysis is not a fast process. However, quick test kits (fig. 1A) are available that can be used to rapidly identify many common diseases in the field.
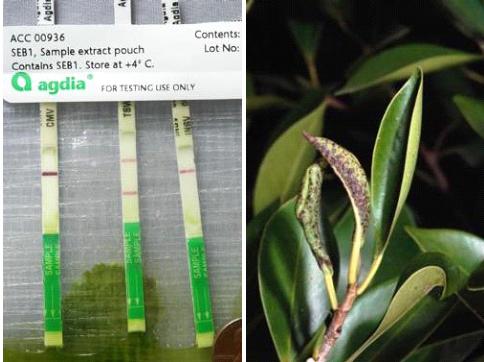
A B
Fig.1. Diagnosing biotic
problems. Plant pathogens can sometimes be rapidly diagnosed using
commercially available quick tests, such as these test strips for
viruses (A). Arthropod pests such as Cuban laurel thrips (shown here on Ficus) cause feeding damage, which can help in pest identification (B). Photos: S.T. Koike (A), J. K. Clark (B).
It is worthwhile to emphasize that diagnosing plant diseases requirescareful examination of the entire plant specimen. Symptoms on leaves, stems, or other above ground plant parts might lead one to suspect that afoliar pathogen is involved. However, these symptoms could also resultif the roots are diseased. Therefore, it is important to conduct a
complete examination of the symptomatic plant.
Because biotic diseases are caused by living microorganisms, the collecting and handling of samples is particularly critical. Samples that are stored for too long a time after collecting or that are allowedto dry out or become hot (if left inside a vehicle, for example) will sometimes cause the pathogen in the sample to die, making pathogen recovery and identification impossible. Plants that have been diseased for a long time and that are in the late stages of disease development will often be colonized by nonpathogenic saprophytic organisms. If these tissues are collected, it will be difficult to recover the primarypathogen of concern because of the presence of these secondary decay organisms. Root samples should be collected carefully as diseased rootsare sometimes difficult to dig out of the potting mix or soil, are
usually colonized by the pathogen as well as secondary agents, and are very sensitive to high temperatures and drying conditions.
Arthropod and other invertebrate pests. Insects,mites, slugs and snails cause damage while feeding on the plant (fig. 1B). Feeding damage is usually associated by the type of feeding characteristics and mouthparts of the insect or pest. For example, mites and insects such as whiteflies, aphids and mealybugs have tubular sucking mouthparts that suck plant fluids, causing buds, leaves, or flowers to discolor, distort, wilt, or drop. Thrips have rasping mouthparts that result in dried out, bleached plant tissue. Caterpillars, weevils, snails and slugs have chewing mouthparts that
make holes and cuts in foliage or flowers. They can also prune plant parts and sometimes consume entire plants.
If present, these pests are visible with the naked eye, a 10 X hand lens, or stereomicroscope, all depending upon their size. An assessment of whether the identified arthropod or invertebrate matches the plant damage it is associated with must be determined. Sometimes the identified arthropod or invertebrate may not be the sole problem or
could, in fact, be a beneficial organism or insignificant pest.
Aphids, whiteflies, thrips, leafhoppers and some other insects that suck plant juices may vector pathogens such as viruses and phytoplasmas (and to a lesser extent fungi and bacteria). They can feed on infected plants, acquire the pathogen, feed on healthy host plants and transmit the pathogen to the new host. The insects do not necessarily have to bepresent in large numbers to cause a significant disease outbreak. The insect vectors are not always present at the same time the disease symptoms are being expressed.
The excrement and byproducts from these pests can also provide clues that the pests have been or are actively present. Caterpillars and other chewing pests produce dark excrement or droppings. Greenhouse thrips and plant bugs produce dark, watery, or varnish-like droppings onfoliage. Aphids, whiteflies, soft scales, and some other sap-sucking insects excrete excess plant fluids as honeydew, a sticky sap, which provides a medium for the growth of sooty mold.
Diagnosing Abiotic Problems
Nutrient deficiencies and toxicities. Nutrientdeficiencies and toxicities reduce shoot growth and leaf size, cause leaf chlorosis (fig.2A), necrosis and dieback of plant parts. However, nutrient deficiencies cannot be reliably diagnosed on the basis of symptoms alone because numerous other plant problems can produce similarsymptoms. There are general symptoms that can be expressed by deficiencies of nutrients but usually leaf and/or soil samples are
needed to confirm the problem.
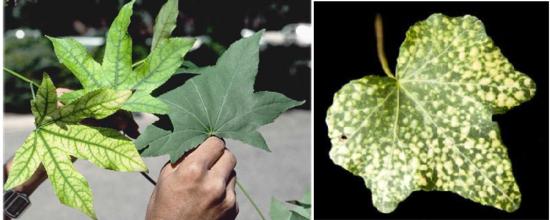
A B
Fig. 2. Examples of abiotic problems. Iron deficiency on sweet gum (Liquidambar styracifolia) showing interveinal chlorosis (A). Chorotic spots on Hedera caused by a miticide application at a higher dosage rate than specified on the pesticide label (B). Photos: E. Martin (A), S. A. Tjosvold (B).
Herbicide, insecticide and fungicide phytotoxicity. Herbicidesused to control weeds in crops or in non-cropped areas sometimes injureornamental crops when they are not used in accordance with label instructions. Examples include when an herbicide is used in or around sensitive non-target crops, when an herbicide rate is increased above tolerable limits, or when an applicator makes a careless application. By understanding the mode of action of the herbicide, one can determine if the symptom fits an herbicide application. Herbicide detection in affected plants is possible with the help of a specialized laboratory but the analysis can be expensive. To minimize the cost of testing, the laboratory will need to know the suspected herbicide or its chemical group to narrow the analysis. Pesticides and fungicides occasionally cause obvious plant damage.
Symptoms can vary widely. Generally, flower petals are more susceptible to damage from pesticide applications than are leaves. The younger and more tender the leaves the more susceptible they are to pesticide applications. Hot weather can exacerbate the damage the chemicals cause. Pesticides that have systemic action can have a more profound effect. Some active ingredients can adversely affect the photosynthetic mechanism or other physiological processes and can resulti n a general leaf chlorosis, interveinal chlorosis, leaf curling and stunting. Emulsifiable concentrate (EC) formulations, soaps and oils can adversely affect the waxy surface layer that protects the leaf from desiccation. Applications with these products can result in the loss ofthe shiny appearance of a leaf, leaf spotting and necrosis. Pesticidesapplied as soil drenches can cause poor germination, seedling death, or
distorted plant growth.
Check label precautions against use on certain species. Make sure thepesticide is not applied more frequently or at a higher rate (fig. 2B) than recommended, or that the pesticide is not mixed with incompatible pesticides. When in doubt as to whether the plant species is sensitive to the pesticide, spray a few plants and observe them for several days to a week for any signs of damage before spraying any more of the plants.
Physiological and Genetic Disorders
There are numerous disorders that can occur because of environmental extremes — too much or too little of an environmental element such as light, temperature, water, or wind. Sunburn is damage to foliage and other herbaceous plant parts caused by a combination of too much light and heat and insufficient moisture. A yellow or brown area develops on foliage, which then dies beginning in areas between the veins. Sunscaldis damage to bark caused by excessive light or heat. Damaged bark becomes cracked and sunken. Frost damage causes shoots, buds and
flowers to curl, turn brown or black and die. Hailstones injure leaves,twigs, and in serious cases even the bark. Chilling damage in sensitive plants can cause wilting of foliage and flowers and development of dark water-soaked spots on leaves that can eventually turn light brown or bleached, and die. Physical and mechanical injury can occur when plants are mishandled during transport or routine cultural practices. Wounds might serve as entry sites for plant pathogens and can attract boring insects to woody stems.
In closed environments such as greenhouses and nursery storage areas,plants can be exposed to toxic levels of ethylene gas. Sources of ethylene include improperly functioning or unvented greenhouse heaters; exhaust from engines of forklifts and vehicles; cigarette smoke; damaged, decaying, or dying plants; and ripe or decaying fruit. Toxic levels of ethylene gas can cause premature abscission of flower buds, petals (fig. 3) and leaves. Other symptoms include wilted flowers, chlorosis, twisted growth or downward bending of stems and leaves and undersized or narrow leaves.
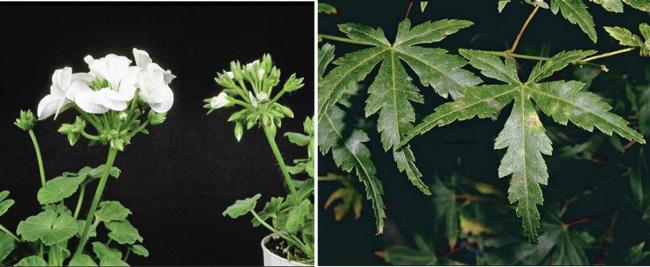
A B
Fig. 3. Poor air quality can
lead to physiological disorders. Shattering (petal drop) on geranium was
caused by plant exposure to low levels of ethylene in the greenhouse or
during postharvest storage (A). Yellowish and brownish patches on
Japanese maple leaves are damage caused by ozone (B), an outdoor air
pollutant. Photos: J. K. Clark.
Outdoors, exposure of nursery plants to air pollutant gases such as ozone (fig. 3), carbon monoxide, nitrous oxides and sulfur dioxide can cause damage. Typical symptoms vary widely, but include slow growth anddiscolored, dying, or prematurely dropping foliage. Damage is often found where plants are located near sources of polluted air such as near
freeways or industries or where weather and topography concentrate the pollutants.
Sometimes plants or plant shoots exhibit an unusual and sudden changeof color producing discrete markings of variegation. For example, a plant with entirely green leaves suddenly produces a shoot that has leaves with edges lacking green pigment, stripes, or blotches. A new shoot such as this is probably a chimera (fig. 4). It is produced when a genetic mutation occurs in a specific region of the growing tip resulting in a section with genetically different cells. The ostensible result of the genetic change is dependent on the arrangement of the genetically different cells in the shoot tip and their expression. This can lead to sometimes bizarre variegation forms or sometimes forms thatare quite desirable. Sometimes variegation can be caused by viruses. Viruses usually cause non-uniform chlorosis, such as mosaics, while
chimeras usually produce patterned forms such as variegation of color onleaf margins, stripes, or complete loss of pigment. Some viroids may also cause bleaching of pigments in leaves; such symptoms, however, are generally produced throughout the plant and are not restricted to a single shoot. Some nutrient disorders can cause variegation but these disorders usually do not arise from a specific shoot as with chimeras.
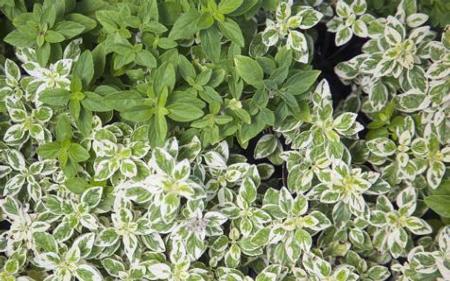
Fig. 4. Genetic disorder.
Growing points with variegated leaves can sometimes arise spontaneously
from some species such as this Origanum. Genetic variants such as this are sometimes confused with plants with virus disease or nutrient deficiency symptoms. Photo: S. A. Tjosvold.
Steve Tjosvold is Environmental Horticulture Advisor and
Steve Koike is Plant Pathology Farm Advisor, UC Cooperative Extension,
Santa Cruz and Monterey counties.
This article was condensed from: Diagnosing Plant
Problems, Chapter 11. In Newman, J. (ed) Container Nursery Production
and Business Management. Univ. of Calif. Agric. and Nat. Resources.
Publication 3540. Richmond, CA.
References
Boxer P, Sandmann G. 1989. Target sites of herbicide action. Boca Raton, FL: CRC Press.
Costello L, Perry E, Matheny N, Henry M, Geisel P. 2003. Abiotic
disorders of landscape plants: A diagnostic guide. Oakland: University
of California Division of Agriculture and Natural Resources Publication
3420.
Derr JF, Appleton BL. 1988. Herbicide injury to trees and shrubs: A
pictorial guide to symptom diagnosis. Virginia Beach, VA: Blue Crab
Press.
Dreistadt SH. 2001. Integrated pest management for floriculture and
nurseries. Oakland: University of California Division of Agriculture and
Natural Resources Publication 3402.
Eagle, DJ. 1981. Diagnosis of herbicide damage to crops. New York, NY: Chemical Publishing Co.
Grogan RG. 1981. The science and art of plant disease diagnosis. Annual Review of Phytopathology 19:333–351.
Ratzinger EJ, Mallory-Smith C. 1997. Classification of herbicides by
the site of action for weed resistance management strategies. Weed
Technology 11:384–393.
Schubert TS, Breman LL. 1988. Basic concepts of plant disease and how
to collect a sample for disease diagnosis. Plant Pathology Circular No.
307. Florida Department of Agriculture and Consumer Services, Plant
Pathology Circular No. 307.
Sharma MP. 1986. Recognizing herbicide action and injury. Alberta Environmental Centre, Alberta Agriculture. Agdex 641–647.
Shurtleff MC, Averre CW. 1997. The plant disease clinic and field
diagnosis of abiotic diseases. St. Paul, MN: American Phytopathological
Society Press.
Stewart TM, Galea VJ. 2006. Approaches to training practitioners in
the art and science of plant disease diagnosis. Plant Disease
90:539–547.
Tickes B, Cudney D, and Elmore C. 1996. Herbicide injury symptoms.
Tucson, AZ: University of Arizona Cooperative Extension Publication No.
195021.
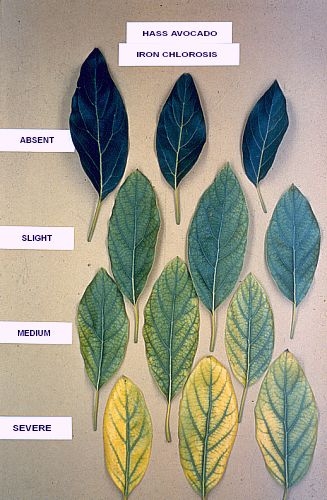