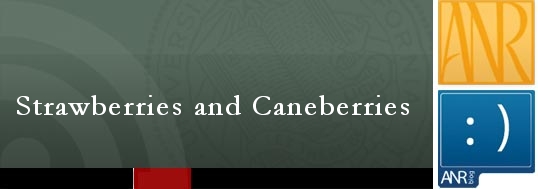
- Author: Mark Bolda
Some points to ponder on a rainy few days regarding phosphorous fertility in strawberries.
Since the work described below was conducted both years in a soil of or above 80 ppm phosphorous, we can’t define where the agronomic threshold for crop response from additional phosphorous lies and subsequently can’t publish in a peer reviewed journal. Subsequently this is not meant to be a guide for phosphorous fertility in California strawberries. Nevertheless, since many of the soils we farm are well within the concentrations of P described, this work could be instructive to those thinking about soil fertility in strawberries.
Introduction: There is a significant body of work in lettuce and several other vegetable crops on the California Central which finds that above a certain soil test threshold concentration there is no longer any plant response to any added phosphorous (P). The following two year study was designed to approach the hypothesis that this also holds true for strawberries.
Materials and Methods:
Year 1 (2008-2009): The field for the study in year one was located south of Salinas and had the following characteristics:
OM |
Sand |
Silt |
Clay |
PPM Olsen P |
2.5% |
49 |
26 |
25 |
90 |
The area for experiment had 24-0-15 added on 9/23/08 at the rate of 362 lb/A applied. Plots to contain phosphorous had super phosphate applied to them at the rate of 48 lb P/A.
Supplemental fertilizer applications to trial plots through the season were in total the equivalent of 54.7 lb N, 60 lb P, and 60 lb K and were achieved with applications of 3-18-18, 20-20-20, and ammonium sulfate.
Yield data for marketable and cull fruit was taken once a week beginning April 7 and ending September 16. As soil phosphorous levels do not change rapidly even in the presence of a crop, soil data for phosphorous concentration was taken once from the field before trial placement and then on August 24, 2009, and plant tissue data consisting of petioles and blades was taken once a month beginning in January, 2009.
Year 2 (2009-2010):
Field for year 2 was near to the field for year 1, so initial soil characteristics were similar.
The area for the experiment had 325 lb/A of 27-0-18 applied to the plots free of phosphorous addition and 24-8-18 at an equivalent rate to the above applied to plots with phosphorous. The result for pre-plant fertilizer addition then was 78 lb N, 26 lb P, and 49 lb K applied to phosphorous addition plots and the same amount of nitrogen and potassium applied to plots without added phosphorous.
Supplemental fertilizer applications to trial plots through the beginning of September were in total the equivalent of 172 lb N, 0 lb P and 12 lb K and were achieved with applications of CN9, 0-0-25, and UN32.
Yield data for marketable and cull fruit was taken once a week beginning April 30 and ending September 2. Soil data was taken once a month starting in October, 2009 and no samples taken in June or July of 2010. Plant tissue data consisting of whole leaf blade nutrient concentrations was taken April, May, August and September.
Results- Year 1
Fruit Yield: There were no significant differences in marketable fruit yield or number in any month sampled, nor were there any significant differences in yields and numbers of cull fruit.
Blade and petiole information given below is a summary of the data sampled over the course of the first year of this study. There are no significant differences by date between plots with P added and those with no P added.
Petioles by sampling date
|
NO3 ppm |
PO4 ppm |
%K |
3/2/2009 |
|
|
|
P added |
2295 |
2000 |
2.45 |
No P added |
2310 |
2035 |
2.55 |
5/1/2009 |
|
|
|
P added |
2576 |
1830 |
2.71 |
No P added |
2692 |
1690 |
2.56 |
8/24/2009 |
|
|
|
P added |
1237.5 |
1657.5 |
2.01 |
No P added |
1137.5 |
1690.0 |
1.89 |
Blades by sampling date
|
%N |
%P |
%K |
1/13/2009 |
|
|
|
P added |
3.52 |
0.74 |
2.31 |
No P added |
3.54 |
0.64 |
2.21 |
3/2/2009 |
|
|
|
P added |
3.62 |
0.84 |
2.06 |
No P added |
3.67 |
0.89 |
2.10 |
5/1/2009 |
|
|
|
P added |
2.87 |
0.41 |
1.71 |
No P added |
2.90 |
0.39 |
1.81 |
8/24/2009 |
|
|
|
P added |
2.64 |
0.36 |
1.76 |
No P added |
2.63 |
0.35 |
1.69 |
Field soil phosphorous was 90 ppm as per Olsen’s P before trial placement and average readings were 85 ppm from plots with additional phosphorous added, and 87 ppm from plots where no phosphorous was added. These readings are not significantly different from one another.
Results- Year 2
Fruit yield: There were no significant differences in marketable fruit yield or number in any month sampled, nor were there any significant differences in total yield of cull fruit or number.
Blade and soil nutrient information given below is a summary of the data sampled over the course of the second year of this study. Unless indicated so, there are no significant differences between plots with P added and those with no P added by date.
Blades by sampling date
|
%N |
%P |
%K |
4/8/2010 |
|
|
|
P added |
2.58 |
0.56 |
1.6 |
No P added |
2.62 |
0.55 |
1.6 |
5/27/2010 |
|
|
|
P added |
2.72 |
0.36 |
1.56 |
No P added |
2.74 |
0.37 |
1.54 |
8/10/2010 |
|
|
|
P added |
2.46 |
0.34 |
1.38 |
No P added |
2.50 |
0.34 |
1.41 |
Soil by sampling date
|
NO3 ppm |
Olsen P |
K ppm |
4/8/2010 |
|
|
|
P added |
16.35 |
85 |
179.3 |
No P added |
16.15 |
83 |
175.8 |
5/27/2010 |
|
|
|
P added |
10.50 |
86 |
170.3 |
No P added |
3.51 |
76 * |
182.9 |
8/10/2010 |
|
|
|
P added |
9.73 |
84 |
106.4 |
No P added |
9.91 |
82 |
125.5 |
* Significantly differs (P=0.05, Student Newman Kuels)
Comments and Discussion: It is clear that the addition of phosphorous in this study covering two years of distinct strawberry crops did not result in yield differences, nor in plant tissue concentrations.
That soil P values did not decline very much, if at all, in both years should not be surprising. For one, the soil test value is a measure of the soil P equilibrium, which means that when plants remove P from the soil solution, additional P comes into the solution from precipitated forms. In other words, a soil with a high P test like the one in these tests simply backfills what is taken up by the plant from its ample reserve. Secondly, according to sampling done by Tim Hartz and his lab over the past few years, we have no indication that strawberries are removing more than 40 lb P per acre per season, meaning that soil test P would not decline more than 15 ppm even if no more P were to come into solution as described above. It simply will not fall very much.
Many thanks to Tim Hartz at UC Davis and the DANR Analytical Laboratory at Davis for their dedicated assistance with this work.
Finally, I want to thank the grower who collaborated with me on this work for two years. You know who you are, and you helped me a lot- I wouldn’t have been able to even think about doing this study if you hadn’t helped me so much. Thank you!
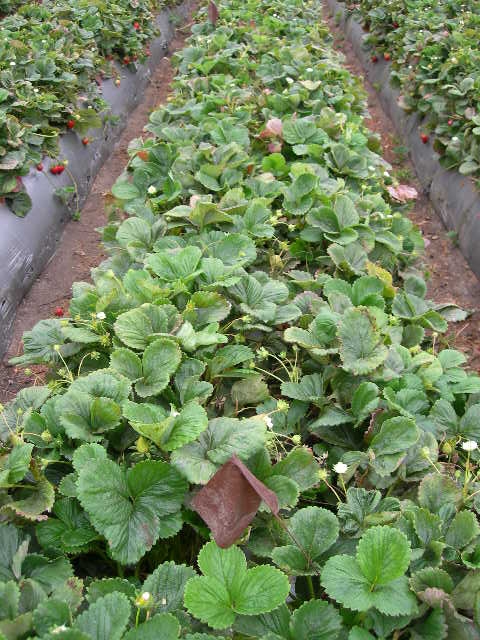
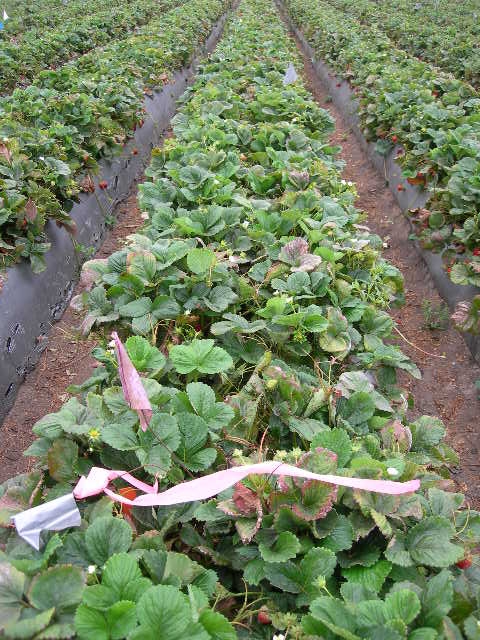
- Author: Mark Bolda
- Author: Tom Bottoms
- Author: Tim Hartz
It has been more than 30 years since UC published strawberry leaf nutrient diagnostic guidelines (Publication 4098, ‘Strawberry deficiency symptoms: a visual and plant analysis guide to fertilization’, released in 1980). In the years since that publication, varieties, production practices and yield expectations have changed considerably. In 2010 we began a project, funded by the California Strawberry Commission, to reevaluate leaf and petiole nutrient sufficiency ranges for day-neutral strawberries. With the cooperation of many berry growers in the Watsonville-Salinas and Santa Maria areas we collected leaf and petiole samples from more than 50 ‘Albion’ fields over the past two production seasons. In each field samples were collected 5 times over the production season, from early spring through September, to document the nutrient concentration trends from pre-fruiting to post-peak production. Leaf samples were analyzed for total concentration of nitrogen (N), phosphorus (P), potassium (K), calcium (Ca), magnesium (Mg), sulfur (S), zinc (Zn), manganese (Mn), iron (Fe) and copper (Cu). Petioles were analyzed for NO3-N, PO4-P and K concentration.
After the season cooperating growers provided yield information, which allowed us to categorize the fields as being ‘high yield’ or 'low yield’. We then applied a process called DRIS (Diagnosis and Recommendation Integrated System) to mathematically evaluate the difference in nutrient concentrations as well as nutrient ratios between high yield and low yield fields. This process allowed us to identify which of the high yield fields were ideally balanced nutritionally. From this group of nutritionally balanced, high yield fields we were able to calculate a DRIS sufficiency range for each nutrient at each growth stage.
Fig. 1 shows that leaf N, P and K concentrations were highest before harvest began (stage 1, which was late February in Santa Maria and late March in Watsonville-Salinas), and declined to a reasonably stable level throughout the main harvest period (stages 3-5, May-July in Santa Maria, June-August in Watsonville-Salinas). The decline in leaf macronutrient concentrations during the peak harvest period was expected; it happens in many fruiting crops because the leaves rapidly translocate nutrients to the developing fruit. By contrast, micronutrient concentrations either increased from early vegetative growth to the main harvest period (as was the case for B, Ca and Fe), or remained reasonably stable over the entire season (all other micronutrients). The vertical bars on each data point on Fig. 1 indicate the range of values typical of nutritionally balanced, high yield fields at each growth stage. These are the DRIS sufficiency ranges; leaf nutrient concentrations within these ranges can safely be assumed to be adequate for high yield production.
Table 1 lists the DRIS leaf nutrient sufficiency ranges for pre-harvest and main harvest growth stages. For the sake of comparison, both the sufficiency ranges given in UC Publication 4098 and the current University of Florida guidelines have been included. Although for most nutrients the ranges match pretty well, for others there are substantial differences. Where the DRIS sufficiency range is substantially higher than the other sources (Ca, Mn and Fe, for example) it is because those nutrients are in such abundant supply in our coastal soils that plant uptake is far in excess of actual plant requirement; for those nutrients a lab test result marginally below the DRIS range would not be a matter of concern.
For several nutrients (N, Zn and Cu) the DRIS sufficiency range fell below the other recommendations. We are confident that the DRIS ranges represent nutrient sufficiency because they were determined by measuring the levels common in high yield fields. The field survey approach used in this project ensured that a wide range of field conditions and grower practices were included, so the results are broadly representative of the coastal industry. Also, for all three nutrients the average leaf concentrations of the high yield and low yield groups were essentially equal, suggesting that availability of these nutrients did not limit yields.
Fig. 2 shows the trends in petiole nutrient concentrations over the season. Petiole NO3-N was so highly variable as to be nearly worthless as a diagnostic technique; during peak fruit harvest (our sampling dates 3 and 4) petiole NO3-N in high yield fields varied from < 200 PPM to 2,600 PPM. While we believe that leaf total N is a more reliable measurement, this study suggests that maintaining petiole NO3-N > 1,000 PPM pre-harvest, and > 400 PPM during peak harvest, is adequate to maintain high productivity. Given the high variability of petiole NO3-N it is possible that concentrations < 400 PPM would be adequate during the summer.
Petiole PO4-P and K were less variable than petiole NO3-N. Maintaining PO4-P > 1,200 PPM throughout the season should ensure P sufficiency. Given the high soil P availability in most coastal soils rotated with vegetable crops, this level is probably much higher than the ‘critical value’. Maintaining petiole K > 2.5% preharvest, and > 1.5% during peak harvest, appears to be adequate.
Table 1. Comparison of DRIS leaf nutrient sufficiency ranges with prior UC recommendations, and current University of Florida guidelines.
|
|
Nutrient sufficiency ranges |
||
Growth stage |
Nutrient |
DRIS |
UC Pub. 4098 |
University of Florida |
Pre-harvest |
% N |
3.1 - 3.8 |
|
3.0 - 3.5 |
|
% P |
0.50 - 0.90 |
|
0.20 - 0.40 |
|
% K |
1.8 - 2.2 |
|
1.5 - 2.5 |
|
% Ca |
0.6 - 1.3 |
|
0.4 - 1.5 |
|
% Mg |
0.33 - 0.45 |
|
0.25 - 0.50 |
|
% S |
0.19 - 0.23 |
|
0.25 - 0.80 |
|
PPM B |
31 - 46 |
|
20 - 40 |
|
PPM Zn |
13 - 28 |
|
20 - 40 |
|
PPM Mn |
75 - 600 |
|
30 - 100 |
|
PPM Fe |
70 - 140 |
|
50 - 100 |
|
PPM Cu |
3.3 - 5.8 |
|
5 - 10 |
|
|
|
|
|
Main harvest |
% N |
2.4 - 3.0 |
> 3.0 |
2.8 - 3.0 |
|
% P |
0.30 - 0.40 |
0.15 - 1.30 |
0.20 - 0.40 |
|
% K |
1.3 - 1.8 |
1.0 - 6.0 |
1.1 - 2.5 |
|
% Ca |
1.0 - 2.2 |
0.4 - 2.7 |
0.4 - 1.5 |
|
% Mg |
0.28 - 0.42 |
0.3 - 0.7 |
0.20 - 0.40 |
|
% S |
0.15 - 0.21 |
> 0.10 |
0.25 - 0.80 |
|
PPM B |
40 - 70 |
35 - 200 |
20 - 40 |
|
PPM Zn |
11 - 20 |
20 - 50 |
20 - 40 |
|
PPM Mn |
65 - 320 |
30 - 700 |
25 - 100 |
|
PPM Fe |
85 - 200 |
50 - 3,000 |
50 - 100 |
|
PPM Cu |
2.6 - 4.9 |
3 - 30 |
5 - 10 |
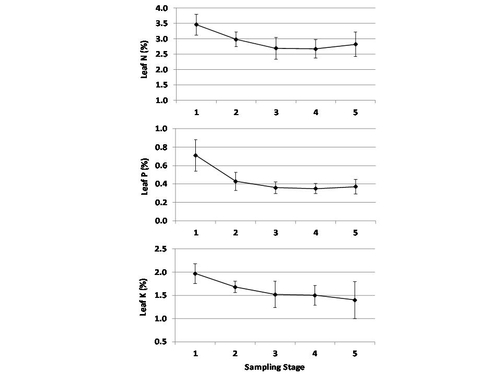
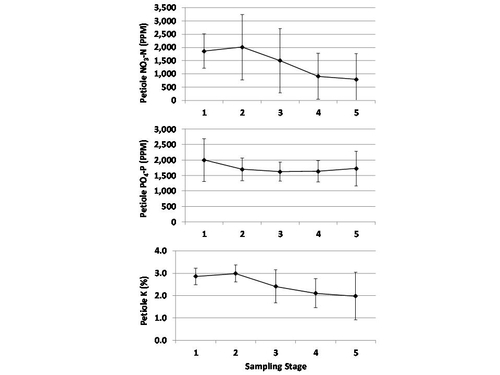
- Posted By: Mark Bolda
- Written by: Mark Bolda
It is at times perhaps tempting to take an uncomplicated view of nutrient deficiency in strawberry. The mantra goes a little bit like yellow leaves are nitrogen deficient, phosphorous deficiency is given by purple leaves, potassium shortages easily marked by scorched leaves and so on.
I don’t believe any of this is so simple and so attempt to challenge this facile thinking when I have the opportunity to do so.
The following situation was a good one. A smallish field of ‘Albion’ variety strawberry was not given supplemental fertilizer beyond a standard dose of preplant slow release 18-8-13 in the area of 500 lbs per acre. The result in late June was as seen below- severely chlorotic plants with a strong tendency towards purpling of the middle age to older leaves. Additionally, flower production had nearly ceased and fruit was sizing down substantially.
I tested the soil to 6 “down at six different points throughout the field, amalgamated them and got the following results:
Nutrient Sampled |
Concentration (PPM) |
Nitrate (NO3) |
4.1 |
Ammonium (NH4) |
3.5 |
Phosphorous |
58 |
Soil pH was a perfectly normal 6.4. As one can see from the numbers above, plant available nitrogen sources nitrate and ammonium were sort of low (usually want to be 10 ppm for either one), while phosphorous was a quite sufficient 58 ppm.
Tissue samples consisted of leaf blades. Four separate samples were taken from different sections of the field and each sample consisted of a dozen leaflets of middle age- meaning not the very old ones at the bottom of the plant nor the young tender leaves emerging from the center of the crown.
Nutrient Sampled |
Average Concentration |
Nitrogen (N) |
1.7 % |
Phosphorous (P) |
0.2025 % |
Potassium (K) |
1.38 % |
Calcium (Ca) |
1.475 % |
Magnesium (Mg) |
0.3675 % |
Sulfur (S) |
0.1325 % |
Copper (Cu) |
4.3 ppm |
Zinc (Zn) |
15 ppm |
Iron (Fe) |
212.5 ppm |
Manganese (Mn) |
805 ppm |
Boron (B) |
79.25 ppm |
Comparing the nitrogen results from the leaf blade with the 2.6-2.8% concentrations described as sufficient as from UC Publication 4098 and Dr. Tim Hartz’s work last year, we see that this vital nutrient is a full percentage point below what is deemed necessary for normal production. Phosphorous, while below the 0.36% field average taken by Dr. Hartz’s study last year, is still understood to be well above the 0.10 % baseline given by UC Publication 4098. All other nutrients, with the exception of zinc (15 ppm compared to a recommended 18-20 ppm), in this sample are well within sufficiency ranges.
Why then the pronounced purpling of the leaves if the phosphorous is not dramatically, if at all, insufficient in the tissue? There are in fact other possibilities. A lack of nitrogen, which is a component of some amino acids and other compounds, can result in a similar build up of excess carbohydrates as in phosphorous deficiency. Essentially, both deficiencies have the same result then that these carbohydrates can end up being used in anthocyanin synthesis resulting in accumulation of these flavonoid pigments. Some are red, others pink and some purple. This may be an explanation why strawberry leaves lacking in nitrogen but not ostensibly lacking in phosphorous are actually turning red to purple.
So, while undoubtedly light green to yellow leaves are still a good indication of nitrogen deficiency in strawberry, don't be fooled that the purple that often accompanies it is actually caused by something else like a lack of phosphorous.
Thank you to Soil Control Lab in Watsonville for processing and evaluating these samples.
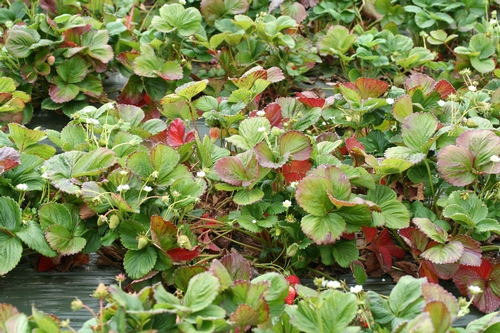
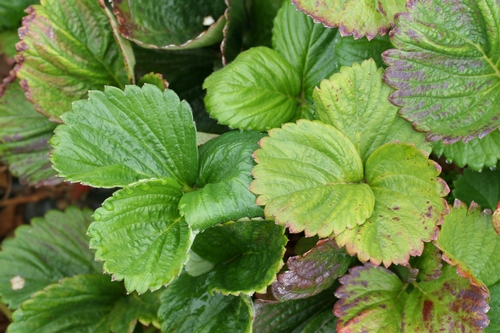
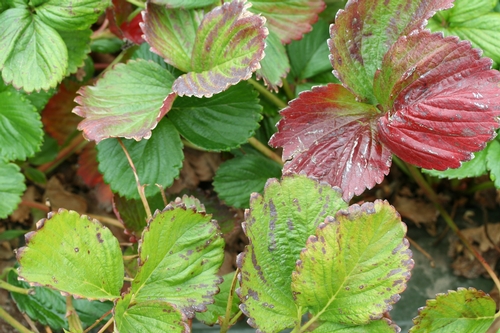
- Posted By: Mark Bolda
- Written by: Mark Bolda
One of the less well understood issues in our industry on the Central Coast is the phenomenon of yellowing of strawberry plants in certain areas of the district, especially in a number of fields north of Salinas. The following is meant to share what we have found out on this problem so far, and discuss some of my thoughts about the most probable cause.
While there are many causes of yellowing in strawberry plants, for example lack of nitrogen, iron or zinc, the yellowing of strawberry plants in the Salinas area seems to stem from something else and occurs in the same area, year after year. In fact, some spots no more than a few meters square give the same symptoms every time strawberries are planted there. Yet, subsequent plantings of other crops such as broccoli or lettuce do not show any yellowing.
To address the thought that the yellowing comes from nutritional deficiency, I have taken many samples with colleagues of these yellow plants and never found anything exceptional nutrient wise. Consider the table below which is an eightfold replicated comparison taken in a large strawberry field south of Castroville with large areas of yellow plants in a field of healthy green plants:
Nutrient |
Healthy Green Plant |
Yellow Plant |
Total Nitrogen (%) |
2.51 |
2.68 |
Total Phosphorous (%) |
0.33 |
0.40 |
Potassium (%) |
1.34 |
1.74 |
Total Sulfur (ppm) |
1830.83 |
2131.25 |
Total Boron (ppm) |
45.54 |
53.50 |
Total Calcium (%) |
1.67 |
1.91 |
Total Magnesium (%) |
0.48 |
0.56 |
Total Zinc (ppm) |
14.63 |
16.50 |
Total Manganese (ppm) |
185.58 |
368.25 |
Total Iron (ppm) |
237.67 |
227.75 |
Total Copper (ppm) |
3.10 |
4.78 |
Soil pH |
7.5 |
7.5 |
What one immediately sees from the table above is that the trend is actually for yellow plants to have HIGHER levels of essential nutrients than their apparently healthier counterparts.
Interestingly, manganese is very much higher, and a t-test tells us significantly so, in the yellow plants than in green plants.
So, the assumption that nutrient deficiencies are leading to this yellowing of the plants is not backed by the evidence of a plant tissue test. To be sure, yellowing from nitrogen tends to be stronger on the outer, older leaves as this mobile nutrient is transported to the younger leaves. Deficiency of zinc generally has a green halo around the leaf edges. Perhaps the symptoms are consistent with that of iron deficiency, and indeed the iron from the soil sample from around the plant itself is significantly higher around the green plant than the yellow. However, the levels of iron in the evaluated plants are well above those described as critical by UC Publication 4098 and Tim Hartz’s strawberry fertility work in 2010.
One of the considerations though all of this research is that the yellowing is caused by waterlogging and a subsequent deficiency in the amount of oxygen available to the plant. This is not necessarily water or saturation that is easily measurable at the surface and may be deeper down in the bed. It is also possible that salinity, which has a slight inverse effect on the solubility of oxygen in water, is also playing a role.
Plants respond to decreased oxygen levels, known as hypoxia, in different ways and some species are in fact quite sensitive to this condition. Roots, as the plant organ which face the hypoxic condition in a waterlogged soil, respond to this stress by switching from respiration to a fermentative metabolism which in turn increases the demand for carbohydrates. That this metabolic change in strawberry is the cause of the yellowing in our strawberries is something which yet remains to be explored.
As a final thought, consider the the fourth picture below in which the drip tape on the right was clogged and less water delivered to that bed for several weeks. The result was a lessening in the yellowing of the plants in that bed, and only that bed. Absolutely, this is not a very scientific evaluation, but it does strongly suggest that excessive water from the plant's perspective has something to do the yellowing we know from around Salinas.
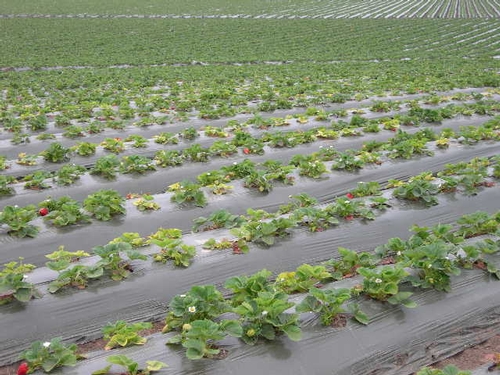
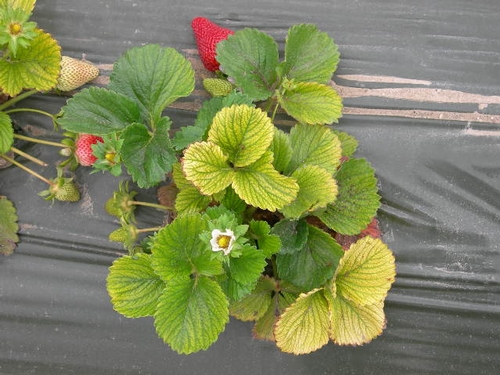
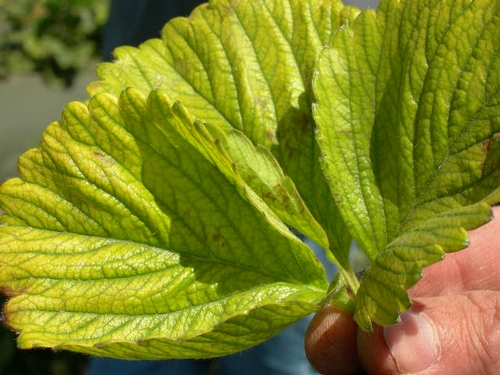

- Author: Mark Bolda
by Tim Hartz and Mark Bolda
During the 2010 production season we conducted a survey of approximately 30 commercial fields in the Watsonville-Salinas area to develop information on strawberry nutrient requirements, and to evaluate current fertilizer practices. We did whole plant sampling in four fields, two planted with ‘Albion’ and two planted with a day-neutral proprietary variety. In all fields the uptake of nitrogen was slow through the winter months; by the end of March total crop N uptake was less than 20 lb/acre. From that point forward, crop N uptake was quite steady, averaging between about 1-1.2 lb N per acre per day. By the end of August, total N uptake ranged between 140-190 lb N/acre, with the higher amounts in higher yield fields. Total phosphorus (P) and potassium (K) uptake averaged about 40 and 230 lb/acre, respectively.
Soil sampling showed that the majority of fields began the season with high soil test levels of P and K, in many cases high enough that P and K fertilization would not be required to produce a good berry crop. However, it appeared that growers often follow a preplant ‘recipe’ when it comes to fertilization, and that typical ‘strawberry mix’ controlled release N/P/K fertilizers were nearly always applied, regardless of soil test nutrient levels. The efficiency of nitrogen applied preplant is also questionable. The N in common preplant controlled release fertilizers becomes available at a relatively steady rate over 6-8 months, meaning that if applied in late October more than half the N is likely to be released by the end of March. Since crop N uptake by the end of March is less than 20 lb/acre, any additional fertilizer N released by that time will be subject to leaching by rain or irrigation. A moderate amount of preplant controlled release N provides a guarantee of some N availability during the winter, but a large fall application is likely to be inefficient.
Most of the growers we worked with provided us with their fertilization records. On average, they applied a seasonal total of 187 lb N/acre, about evenly split between preplant and fertigated N. However, there were large differences among growers in their N management; seasonal rates varied from less than 150 lb N/acre to nearly 300 lb N/acre, with some growers using nearly all preplant N, while others applied nearly all N through fertigation. We found no correlation between seasonal N rate and fruit yield.
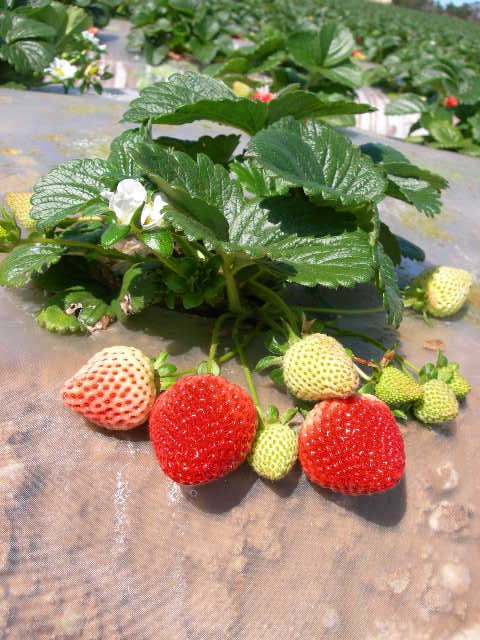